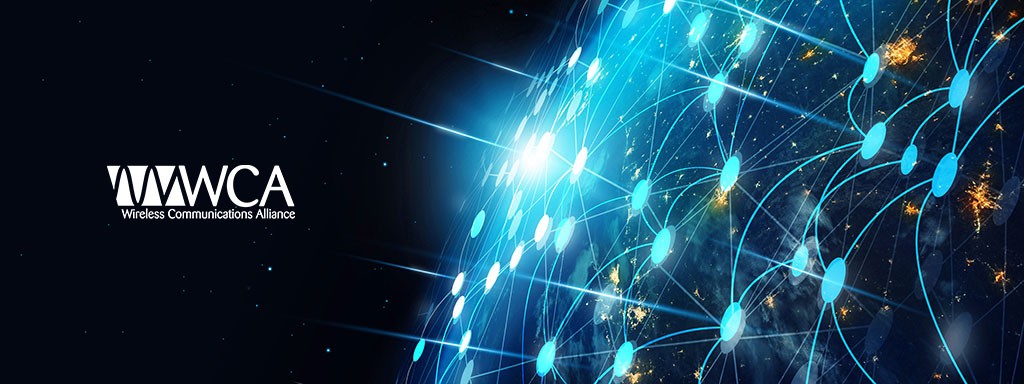
Wireless and Connected Technology Explained – We explore the complexities of connected technology. We provide insightful analyses, delve into current trends, and forecast future developments. Whether you are tech-savvy or simply curious, our journey through 5G, IoT, and network innovations will enlighten you. Welcome to our detailed examination of connected tech, where we explore 5G/6G, IIoT, Edge computing. Connected AI, mmWave, diverse network structures, and groundbreaking technologies are shaping our digital era.
4G (1)
4G LTE Cat-1bis modules are a type of wireless communication module designed for the LTE (Long-Term Evolution) network. They are an enhancement of the original Category 1 (Cat-1) LTE modules and offer some specific features and improvements. Here are the key aspects of 4G LTE Cat-1bis modules:
- Enhanced Data Rates: While standard Cat-1 modules support data rates up to 10 Mbps for download and 5 Mbps for upload, Cat-1bis modules are designed to provide improved data rates. The exact speeds can vary, but they are generally higher than the basic Cat-1 specifications.
- Power Efficiency: Cat-1bis modules are designed to be more power-efficient compared to their predecessors. This makes them suitable for IoT devices that require a balance between moderate data rate requirements and long battery life.
- Lower Complexity: These modules are less complex than higher category LTE modules (such as Cat-4 or Cat-6), which makes them a cost-effective solution for applications that do not require very high data rates.
- Applications: 4G LTE Cat-1bis modules are ideal for a range of IoT and M2M (Machine to Machine) applications that require better connectivity than 2G or 3G but do not necessarily need the high speeds offered by more advanced LTE categories. These include telematics, smart metering, security systems, remote monitoring, and other IoT applications.
- Backward Compatibility: Like other LTE technologies, Cat-1bis modules are typically backward compatible with existing 2G and 3G networks, ensuring connectivity even in areas where 4G coverage is not available.
- VoLTE Support: Some Cat-1bis modules support Voice over LTE (VoLTE), which can be a critical feature for certain applications that require voice communication capabilities.
In summary, 4G LTE Cat-1bis modules provide a balanced solution for IoT and M2M applications, offering enhanced data rates and power efficiency compared to standard Cat-1 LTE modules, without the complexity and cost of higher category LTE technologies.
5G (9)
5G Advanced, often referred to as “5G-Advanced” or “5.5G,” represents the evolution and enhancement of 5G technology. It is considered the next phase in 5G development, following the initial release of 5G standards (Release 15 and Release 16 by the 3GPP). 5G Advanced aims to expand and improve upon the capabilities of 5G to meet growing demands and emerging technological trends. Key aspects of 5G Advanced include:
- Enhanced Performance: 5G Advanced aims to further increase data rates, reduce latency, and improve network efficiency beyond the initial specifications of 5G.
- Improved Network Capacity and Coverage: It focuses on enhancing network capacity to support an even larger number of connected devices, as well as improving coverage, particularly in challenging environments.
- Advanced Network Features: This includes more advanced forms of network slicing, improved Massive MIMO (Multiple Input Multiple Output) technologies, and enhancements in beamforming for better signal direction and strength.
- Integration with Emerging Technologies: 5G Advanced is expected to better integrate with technologies like Artificial Intelligence (AI), Machine Learning (ML), and edge computing, offering more intelligent and responsive network solutions.
- Support for Diverse Applications: While 5G already supports a wide range of applications, 5G Advanced will further expand capabilities in areas such as the Internet of Things (IoT), ultra-reliable low-latency communications (URLLC), and enhanced mobile broadband (eMBB).
- Sustainability and Energy Efficiency: A focus on sustainability, with improvements in energy efficiency, is a key aspect of 5G Advanced, addressing the environmental impact of expanding network infrastructures.
- Research and Standardization: 5G Advanced is currently in the research and standardization phase, with industry and academia collaborating to define its features and capabilities.
5G Advanced represents the continuous evolution of 5G networks, aiming to accommodate the ever-increasing demand for data and connectivity and to enable new applications and technologies that require more advanced network capabilities.
5G Convergence refers to the integration and unification of various technologies, services, and network architectures under the umbrella of 5G wireless technology. This convergence aims to create a more seamless and efficient telecommunications ecosystem. Key aspects of 5G Convergence include:
- Unified Network Architecture: 5G Convergence involves integrating different types of networks, such as cellular, Wi-Fi, and satellite, into a unified system. This allows for more efficient resource management and service delivery.
- Integration of Services: Convergence in 5G isn’t just about network technologies; it also includes the integration of various services like voice, data, and multimedia, providing a comprehensive and seamless user experience.
- IoT and Industrial Integration: 5G Convergence is crucial in the integration of IoT devices and industrial applications, enabling seamless communication between a vast array of devices and systems.
- Network Slicing: A key feature of 5G, network slicing allows the creation of multiple virtual networks over a single physical network infrastructure. This enables the tailored provisioning of network resources for different applications and services.
- Enhanced Data Processing: With the convergence of edge computing and 5G, data processing becomes more efficient. Data can be processed closer to where it is generated, reducing latency and improving response times.
- Support for Diverse Applications: 5G Convergence supports a wide range of applications, from high-speed mobile broadband to mission-critical communication and massive IoT deployments.
- Standardization and Interoperability: Ensuring interoperability and compliance with global standards is essential in 5G Convergence, to enable seamless communication across devices and networks.
- Advanced Technologies Synergy: 5G Convergence brings together advancements like AI, big data analytics, and cloud computing, leveraging these technologies to enhance network performance and user experience.
5G Convergence represents a transformation in how communication networks are built and operated, offering a more integrated, flexible, and efficient approach to meet the diverse demands of modern digital society.
5G Fixed Wireless Access (FWA) is a method of providing wireless broadband internet services to homes and businesses using 5G cellular network technology. It is an alternative to traditional wired broadband like DSL, cable, or fiber optics. Here are key aspects of 5G FWA:
- Use of 5G Technology: 5G FWA utilizes the high-speed and low-latency capabilities of the 5G network to deliver internet services.
- Wireless Connectivity: Unlike traditional broadband that requires physical wiring, FWA uses wireless signals to connect users to the internet. This can significantly reduce the need for extensive physical infrastructure.
- Deployment Ease: FWA is particularly beneficial in areas where laying cables is challenging or not cost-effective. It allows for rapid deployment of broadband services in rural or underserved areas.
- High-Speed Internet: With 5G technology, FWA can offer comparable, and in some cases, superior speeds to wired broadband solutions, suitable for high-bandwidth applications like streaming, gaming, and video conferencing.
- Network Infrastructure: The setup typically involves a 5G modem or router at the user’s location, which communicates with the nearest 5G cell tower to provide internet connectivity.
- Cost-Effectiveness: For network providers, FWA can be a more cost-effective way to expand broadband access, especially in less densely populated areas.
- Improved Capacity and Range: Leveraging advanced 5G technologies like beamforming and Massive MIMO, FWA can offer improved capacity and range compared to earlier wireless technologies.
5G FWA is seen as a key component in the broader rollout of 5G, offering a flexible and efficient way to expand broadband access and bridge the digital divide, particularly in regions where wired infrastructure is lacking or insufficient.
5G Massive IoT refers to the application of 5G technology to massively connect a large number of Internet of Things (IoT) devices. This concept is part of the broader vision of 5G networks, which aim to provide not just faster internet speeds for smartphones but also to enable the interconnectivity of billions of devices. Here are key aspects of 5G Massive IoT:
- High Device Connectivity: One of the primary goals of 5G Massive IoT is to support an extremely large number of connected devices per square kilometer, far exceeding the capacity of previous cellular technologies.
- Low Power Consumption: 5G Massive IoT focuses on providing connectivity to devices that require low power consumption, enabling devices to operate for years on a single battery charge. This is crucial for sensors and devices in remote or hard-to-reach locations.
- Wide Range and Deep Coverage: 5G technology aims to offer enhanced coverage that can reach challenging areas, such as deep indoors or in rural locations, making it suitable for a wide range of IoT applications.
- Small Data Packets: Massive IoT devices typically transmit small amounts of data infrequently. 5G networks are designed to efficiently handle such small data packets, optimizing network usage and performance.
- Diverse Applications: Applications of 5G Massive IoT are diverse and include smart cities, industrial IoT, environmental monitoring, agriculture, smart buildings, and more.
- Integration with Other Technologies: 5G Massive IoT is expected to work in tandem with other technologies like edge computing and AI to process and manage the vast amounts of data generated by IoT devices.
- Enhanced IoT Capabilities: Beyond connectivity, 5G Massive IoT aims to enhance capabilities such as device-to-device communication, real-time data analytics, and automated decision-making processes.
In summary, 5G Massive IoT represents a significant leap in the capability to connect a vast number of IoT devices, enabling new applications and efficiencies across various industries, and is a critical component of the evolving 5G landscape.
5G RedCap (Reduced Capability) is a new feature introduced in the 3rd Generation Partnership Project (3GPP) Release 17. It is designed to cater to devices that require higher capabilities than those offered by LTE-M or NB-IoT (both are narrowband IoT technologies), but do not need the full capabilities of standard 5G devices. This makes 5G RedCap particularly suitable for a specific segment of IoT and industrial applications. Key aspects of 5G RedCap include:
- Lower Device Complexity: 5G RedCap aims to reduce the complexity and cost of devices compared to full-featured 5G devices. This is achieved by scaling down certain aspects of the 5G technology.
- Moderate Data Rates: While offering lower data rates than the full 5G standard, 5G RedCap still provides higher data rates than narrowband IoT technologies, making it suitable for applications that require moderate bandwidth.
- Energy Efficiency: With its reduced complexity, 5G RedCap also aims to improve energy efficiency, which is crucial for battery-powered IoT devices.
- Broad Applications: This technology is ideal for a range of IoT applications, including wearables, industrial sensors, and certain types of smart meters that need more capability than NB-IoT or LTE-M but do not require the high data rates and full capabilities of 5G.
- Network Compatibility: 5G RedCap is designed to be compatible with existing 5G networks, enabling seamless integration with the current infrastructure.
- Balanced Performance: The key advantage of 5G RedCap is its balanced performance, offering better capabilities than narrowband technologies while avoiding the complexity and cost of full 5G.
In summary, 5G RedCap represents an important step in the evolution of 5G and IoT, bridging the gap between narrowband IoT technologies and full 5G, and providing a more cost-effective and efficient solution for a wide range of IoT applications.
CBRS (Citizens Broadband Radio Service) in the context of 5G-Advanced refers to an innovative approach in wireless communication where the CBRS spectrum is utilized for advanced 5G applications. CBRS operates in the 3.5 GHz band (3550 MHz to 3700 MHz) in the United States and is designed to offer a shared spectrum model. This approach is significant in the evolution of 5G networks for several reasons:
- Shared Spectrum Access: CBRS uses a three-tiered shared spectrum access system, allowing for efficient use of the 3.5 GHz band. This system includes Incumbent Access, Priority Access, and General Authorized Access.
- Enhanced Capacity and Coverage: By leveraging the CBRS band, 5G-Advanced networks can enhance capacity and coverage, particularly in densely populated areas or for enterprise use cases.
- Flexibility and Cost-Effectiveness: CBRS offers a more flexible and cost-effective way for organizations to deploy private 5G networks, as it reduces the need for purchasing exclusive spectrum licenses.
- Innovation in Wireless Services: The utilization of CBRS in 5G-Advanced paves the way for innovative wireless services and applications, including IoT deployments, industrial automation, and enhanced mobile broadband.
- Improved Network Performance: The CBRS band is well-suited for 5G use due to its balance between coverage and capacity, making it ideal for a variety of applications from urban to rural deployments.
- Regulatory Framework: The Federal Communications Commission (FCC) has established rules for CBRS, promoting efficient use of the spectrum while protecting incumbent users.
- Compatibility with Existing Technology: CBRS can be integrated with existing LTE and 5G NR technology, allowing for seamless adoption and integration into current network infrastructures.
CBRS 5G-Advanced represents a significant step in diversifying the spectrum usage for 5G, offering new opportunities for network operators, enterprises, and other entities to deploy flexible and efficient 5G solutions.
Enhanced Mobile Broadband (eMBB) is one of the three primary use case categories defined for 5G networks by the 3rd Generation Partnership Project (3GPP), alongside Ultra-Reliable Low-Latency Communications (URLLC) and Massive Machine Type Communications (mMTC). eMBB focuses on providing significantly higher data rates and greater capacity compared to previous mobile network generations. Key aspects of eMBB include:
- High Data Speeds: eMBB aims to deliver peak data rates up to several gigabits per second (Gbps), which is a substantial increase over 4G data rates. This enables applications that require high bandwidth, such as high-definition video streaming, augmented reality, and virtual reality.
- Improved Network Capacity: eMBB is designed to support a higher number of connected devices and higher throughput per area, which is essential for crowded urban areas and for events with high user density.
- Enhanced User Experience: The increased speed and capacity contribute to a significantly enhanced user experience, with faster download and upload speeds, higher quality video content, and more reliable connectivity.
- Broadband Everywhere: eMBB also aims to provide high-speed mobile broadband services in areas where fixed broadband is unavailable or limited, effectively bridging the digital divide.
- Support for Diverse Applications: While eMBB is primarily associated with consumer applications like streaming and gaming, it also supports a wide range of business applications, including cloud services and teleconferencing.
- Advanced Antenna Technologies: The deployment of eMBB involves advanced technologies such as Massive MIMO (Multiple Input Multiple Output) and beamforming, which are key to achieving the high data rates and capacity.
- Spectrum Utilization: eMBB makes use of a wide range of frequency bands, from sub-6 GHz for wide coverage to millimeter-wave bands for high-capacity, short-range coverage.
eMBB represents a significant evolution in wireless broadband capabilities, setting the foundation for a new generation of mobile applications and services enabled by 5G technology.
Massive Machine Type Communications (mMTC) is one of the three main use case categories defined for 5G networks by the 3rd Generation Partnership Project (3GPP), alongside Enhanced Mobile Broadband (eMBB) and Ultra-Reliable Low-Latency Communications (URLLC). mMTC is focused on enabling large-scale communication between devices, typically for IoT applications. Key aspects of mMTC include:
- Large-Scale Connectivity: mMTC is designed to support a vast number of connected devices, potentially in the order of millions per square kilometer. This is crucial for IoT applications that require extensive sensor networks.
- Low Power Requirement: Devices used in mMTC networks are typically designed to be low-power, allowing them to operate for years on a small battery, which is essential for IoT devices deployed in remote or hard-to-reach areas.
- Small Data Packets: mMTC is optimized for the transmission of small, infrequent data packets, which is characteristic of many IoT and sensor applications.
- High Density and Scalability: mMTC networks are designed to handle high device densities, ensuring reliable communication even in environments with a large number of IoT devices.
- Cost-Effective Solutions: The focus is on providing cost-effective connectivity solutions, enabling the deployment of IoT devices and sensors on a large scale without significantly increasing costs.
- Applications: mMTC is applicable in various sectors including smart cities, industrial monitoring, agriculture, environmental sensing, and smart homes, where a large number of devices need to be connected.
- Network Efficiency: Strategies like network slicing are used to efficiently manage and prioritize network resources for mMTC traffic.
mMTC is a key component of the 5G landscape, enabling the widespread and efficient connectivity of IoT devices and facilitating the growth of smart environments and applications.
URLLC, or Ultra-Reliable Low-Latency Communications, is a service category in 5G networks designed to support applications that require very high reliability and extremely low latency. It is one of the three primary use case categories defined for 5G, alongside Enhanced Mobile Broadband (eMBB) and Massive Machine Type Communications (mMTC). Key aspects of URLLC include:
- Low Latency: URLLC aims to achieve end-to-end latency in the order of milliseconds, significantly lower than what is possible in previous generation networks. This is crucial for applications requiring real-time responses.
- High Reliability: URLLC provides highly reliable communication links, with success rates as high as 99.999% for data transmission. This level of reliability is essential for critical applications where errors or delays could have severe consequences.
- Critical Applications Support: URLLC is tailored for use cases such as autonomous vehicles, industrial automation, remote surgery, and other applications where instantaneous, reliable communication is vital.
- Network Slicing: Leveraging network slicing in 5G, specific slices of the network can be allocated for URLLC services, ensuring dedicated resources and prioritization over other types of network traffic.
- Advanced Technologies: The implementation of URLLC involves various advanced technologies, including edge computing, advanced antenna technologies like beamforming, and enhanced modulation techniques to minimize transmission delays and errors.
- Spectrum Efficiency: URLLC requires efficient use of the spectrum to meet its stringent latency and reliability requirements, often using techniques like OFDMA (Orthogonal Frequency Division Multiple Access).
- Standardization: URLLC is part of the 3GPP standards for 5G (starting from Release 15 onwards), which define the technical aspects and requirements for deploying URLLC services.
URLLC is a cornerstone for enabling a wide range of future technologies and applications that depend on rapid, reliable wireless communication, and is a key differentiator of 5G networks from their predecessors.
IoT (4)
The SGP.32 eSIM IoT Technical Specification refers to a set of standards and guidelines developed by the GSMA (Global System for Mobile Communications Association) for the implementation of eSIM technology in IoT (Internet of Things) devices. “eSIM” stands for “Embedded Subscriber Identity Module,” and it represents a significant advancement in SIM card technology. The SGP.32 specification outlines how eSIMs should be integrated and managed within IoT applications. Key aspects of the SGP.32 eSIM IoT Technical Specification include:
- eSIM Profile Management: The specification details how eSIM profiles can be remotely managed and provisioned. This includes downloading, enabling, disabling, and deleting profiles on the eSIM.
- Interoperability: Ensuring that eSIMs and related management systems are interoperable across different manufacturers and network operators is a core focus of the specification.
- Security: SGP.32 includes robust security guidelines for the protection of data on eSIMs. This encompasses secure transmission of eSIM profiles and safeguarding sensitive information.
- Remote Provisioning Architecture for Embedded UICC: The specification provides a detailed framework for the remote provisioning and management of eSIMs in IoT devices, ensuring consistency and reliability in the deployment of eSIM technology.
- Lifecycle Management: It addresses the entire lifecycle of an eSIM, from initial deployment to end-of-life, including updates and maintenance procedures.
- Scalability and Flexibility: The standards are designed to be scalable and flexible to accommodate a wide range of IoT devices and applications, from small-scale consumer products to large industrial systems.
- Integration with IoT Platforms: The specification also considers how eSIM technology integrates with broader IoT platforms and ecosystems, including cloud services and analytics tools.
The SGP.32 eSIM IoT Technical Specification is instrumental in advancing the use of eSIM technology in the IoT space, offering a more flexible, secure, and efficient approach to device connectivity and management.
Narrowband IoT (NB-IoT) is a Low Power Wide Area Network (LPWAN) radio technology standard developed to enable a wide range of devices and services to be connected using cellular telecommunication bands. NB-IoT is one of several standards developed to meet the growing needs of IoT (Internet of Things) applications. Here are some key aspects of NB-IoT:
- Low Power Usage: NB-IoT devices are designed for low power consumption, allowing them to operate for years on a single battery charge. This is ideal for IoT devices that need to be deployed for long periods without maintenance.
- Extended Coverage: NB-IoT provides improved indoor and rural coverage compared to traditional mobile networks. It achieves this by using a simpler waveform that can penetrate deep into buildings and underground areas.
- Narrow Bandwidth: As the name suggests, NB-IoT operates on a narrow bandwidth of just 200 kHz. This narrowband technology is beneficial for applications that require small amounts of data to be transmitted infrequently.
- Cost-Effective: The infrastructure required for NB-IoT is less expensive compared to broader bandwidth cellular networks. This makes it a cost-effective solution for deploying large-scale IoT networks.
- High Connection Density: NB-IoT supports a high number of connected devices per cell. This makes it suitable for applications where many devices need to be interconnected in a condensed area.
- Applications: Typical applications of NB-IoT include smart meters, smart parking, asset tracking, environmental monitoring, and smart agriculture.
- Standardization and Compatibility: NB-IoT is a standardized technology (by 3GPP) and is backed by major telecommunications operators. It is compatible with existing cellular network infrastructure, allowing for easy integration and deployment.
In summary, Narrowband IoT offers a highly efficient, cost-effective, and standardized way to connect a large number of devices over wide areas, making it an integral part of the IoT ecosystem.
The Cellular IoT Ecosystem refers to the comprehensive environment that encompasses technologies, devices, networks, and services enabling cellular connectivity for the Internet of Things (IoT). This ecosystem is built around the use of cellular networks (like LTE, 5G) to connect IoT devices. Here are key components and aspects of the Cellular IoT Ecosystem:
- Cellular Networks: The foundation of this ecosystem is cellular networks, including LTE (4G), 5G, and specialized subsets like NB-IoT and LTE-M, which are designed for low-power, wide-area IoT applications.
- IoT Devices and Sensors: These are the endpoints in the ecosystem, ranging from simple sensors to complex machines. They collect and transmit data over cellular networks.
- Connectivity Management: Tools and platforms that manage and control the connectivity of IoT devices, ensuring seamless communication, security, and data flow.
- Data Processing and Analytics: Once data is transmitted over the network, it is processed and analyzed. This can occur in cloud-based platforms or edge computing devices.
- Applications and Services: The ecosystem is driven by a vast range of applications across various industries such as healthcare, agriculture, smart cities, industrial automation, and more.
- Security: As these devices often collect and transmit sensitive data, security is a crucial component, including encryption, network security protocols, and secure device management.
- Regulatory Framework: Compliance with regional and international regulations and standards is essential for operation within legal and ethical guidelines.
- Service Providers and Ecosystem Partners: The ecosystem involves collaboration between hardware manufacturers, software developers, network operators, service providers, and other stakeholders.
- Innovation and Development: Continuous innovation is key, with ongoing development in areas like 5G technology, low-power wide-area network solutions, and enhanced security protocols.
In summary, the Cellular IoT Ecosystem represents the integration of multiple technologies and components, working together to enable a wide range of IoT applications through cellular connectivity. This ecosystem is evolving rapidly, driven by advancements in cellular technology and the increasing demand for IoT solutions.
Wi-Fi Sensing technology, also known as Wi-Fi positioning or Wi-Fi based sensing, is an innovative use of Wi-Fi signals to detect and interpret movements or changes in the environment. This technology does not rely on traditional video or infrared sensors but uses the characteristics of Wi-Fi signals such as signal strength, phase, and timing. Here are some key aspects of Wi-Fi Sensing technology:
- Movement Detection: Wi-Fi Sensing can detect movement in an environment by analyzing disruptions or changes in Wi-Fi signal patterns caused by motion.
- Location Tracking: It can be used to track the location of devices or people within a Wi-Fi network’s range, based on how their presence affects Wi-Fi signals.
- Privacy-Friendly: Since it doesn’t rely on cameras, Wi-Fi Sensing is considered more privacy-friendly for monitoring and security applications, as it doesn’t capture visual images.
- Smart Home Applications: In smart homes, Wi-Fi Sensing can be used for applications like security alarms, monitoring the well-being of residents, automating lighting or heating based on occupancy, and detecting unusual activities.
- Health Monitoring: It has potential applications in health monitoring, such as fall detection for the elderly or monitoring breathing patterns during sleep.
- Retail and Business Analytics: Businesses can use Wi-Fi Sensing for customer movement and behavior analytics, helping to understand customer preferences and enhance the in-store experience.
- Integration with Existing Hardware: One of the advantages of Wi-Fi Sensing is that it can often be integrated into existing Wi-Fi infrastructure with software updates, reducing the need for additional hardware.
- Emerging Technology: Wi-Fi Sensing is an emerging technology and is continually being developed to improve accuracy, reliability, and the range of applications.
Wi-Fi Sensing technology leverages the widespread availability of Wi-Fi and provides a novel way to gather environmental data without additional hardware, opening up new possibilities in smart environments, security, healthcare, and retail analytics.
LTE (2)
LTE (Long-Term Evolution) networks represent a standard for wireless broadband communication. They are designed to increase the capacity and speed of wireless data networks. LTE is often referred to as 4G LTE and is a major step up from 3G networks in terms of speed and efficiency. Here are key aspects of LTE networks:
- High-Speed Data Transmission: LTE networks provide significantly higher data speeds for both downloading and uploading compared to earlier mobile networks like 3G. This enables faster internet browsing, streaming of high-definition videos, and quicker download times.
- Improved Capacity and Efficiency: LTE networks are more efficient at handling data, voice, and video traffic, leading to more reliable service, even during peak times or in crowded areas.
- Lower Latency: LTE offers reduced latency, which is the time taken for a data packet to travel from its source to its destination. This results in improved performance for applications that require real-time data transmission, like online gaming and video conferencing.
- Enhanced Bandwidth: LTE networks use a wider radio spectrum bandwidth, providing more space for data traffic and thereby improving network capacity and speed.
- Better Coverage: While the extent of coverage depends on the network provider, LTE networks generally provide better and more extensive coverage compared to their 3G counterparts.
- Evolution to LTE-Advanced: LTE-Advanced is an upgrade to the standard LTE technology, offering even higher speeds and capacity. It includes features like carrier aggregation (combining multiple LTE carriers), higher-order MIMO (Multiple Input Multiple Output), and enhanced use of spectrum.
- Global Adoption: LTE is widely adopted around the world, enabling global roaming for LTE-equipped devices, subject to the compatibility of frequency bands between different regions.
LTE networks have been instrumental in driving the growth of mobile internet and are the backbone of modern mobile communication, paving the way for the next generation of wireless technology, including 5G networks.
LTE-Advanced, also known as 4G+, is an enhancement to the original LTE (Long-Term Evolution) technology. It was standardized by the 3rd Generation Partnership Project (3GPP) as part of its Release 10 and beyond. LTE-Advanced aims to provide faster and more efficient data rates, enhanced performance, and better user experience compared to its predecessor, LTE. Key features and improvements of LTE-Advanced include:
- Carrier Aggregation (CA): One of the most significant enhancements in LTE-Advanced. Carrier Aggregation allows the network to combine multiple LTE carriers, boosting data rates by increasing the bandwidth available for data transmission.
- Higher Order MIMO (Multiple Input Multiple Output): LTE-Advanced supports more antennas than LTE, allowing for higher order MIMO configurations. This increases the potential data rate and capacity of the network, especially in densely populated areas.
- Enhanced Use of Spectrum: LTE-Advanced can operate over a wider range of frequency bands and bandwidths, from 1.4 MHz up to 100 MHz. This flexibility enables better use of available spectrum and improves network performance.
- Improved Network Efficiency: Enhanced inter-cell interference coordination (eICIC) and Coordinated Multi-Point (CoMP) operations are introduced to improve network efficiency, especially at cell edges and in densely populated urban areas.
- Advanced Modulation Techniques: LTE-Advanced employs advanced modulation techniques, like 256-QAM (Quadrature Amplitude Modulation), enabling higher throughput under suitable conditions.
- Backward Compatibility: LTE-Advanced is backward compatible with LTE, meaning devices and networks can switch between LTE and LTE-Advanced depending on availability and network conditions.
- Application Scenarios: LTE-Advanced is suitable for high-demand applications such as high-definition video streaming, large-scale online gaming, and high-speed mobile internet access.
In summary, LTE-Advanced represents a significant step forward in mobile network technology, offering increased speed, improved efficiency, and better overall performance, setting the stage for the transition to even more advanced technologies like 5G.
Networks (5)
Brownfield networks refer to existing telecommunications networks that have been previously developed and deployed, often using legacy technologies and equipment. These networks contrast with “greenfield” networks, built from scratch using the latest technologies and standards. Key aspects of brownfield networks include:
- Legacy Systems: Brownfield networks often include older technologies that may not be compatible with the latest standards or innovations. This includes older generations of wireless technology, like 2G and 3G, or traditional wired networks.
- Integration Challenges: Integrating new technologies or upgrades into brownfield networks can be challenging due to compatibility issues with legacy systems and equipment.
- Cost Considerations: While upgrading brownfield networks can be cost-effective compared to building new infrastructure, the process of modernization can be complex and resource-intensive.
- Maintenance and Operations: Maintaining brownfield networks involves managing aging infrastructure, which may require more frequent repairs and upkeep.
- Migration to Newer Technologies: Transitioning from brownfield to more modern network infrastructures, like 4G, 5G, or fiber-optic networks, often requires strategic planning and phased implementation to minimize service disruptions.
- Customer Base: Brownfield networks typically have an existing customer base, which can be an advantage in terms of market presence, but also poses the challenge of ensuring service continuity during upgrades.
- Regulatory Compliance: Ensuring that brownfield networks comply with current regulatory standards is crucial, especially when integrating new technologies or services.
Understanding and effectively managing brownfield networks is essential for telecommunications operators, especially in the context of ongoing industry advancements and the need for digital transformation.
Greenfield networks refer to telecommunications networks built from scratch with no constraints from existing systems or infrastructures. This term is often used in contrast to “brownfield” networks, which involve upgrading or integrating with existing network infrastructure. Key aspects of greenfield networks include:
- Latest Technologies: Greenfield projects offer the opportunity to deploy the latest technologies, such as 5G in wireless networks or advanced fiber optics in wired networks, without the limitations of legacy systems.
- Design and Implementation Flexibility: Building a greenfield network allows for more flexibility in design and implementation, enabling a more optimized and efficient network architecture that is future-proof.
- Cost Considerations: While the initial investment for a greenfield project might be high due to the need for new infrastructure, it can be more cost-effective in the long term due to lower maintenance and operational costs.
- Faster Deployment of Advanced Services: Greenfield networks can more rapidly deploy advanced services and applications, benefiting from the inherent efficiencies and capabilities of the latest technologies.
- Challenges in Market Penetration: For new operators, establishing a greenfield network can be challenging in terms of gaining market share and attracting customers, especially in regions with established competitors.
- Regulatory Compliance: Greenfield projects must comply with all current regulatory standards and requirements, which can vary depending on the region and the type of services offered.
- Sustainability and Environmental Considerations: New network deployments can incorporate sustainability practices and eco-friendly technologies from the outset.
Greenfield networks represent an ideal scenario for deploying the most advanced telecommunications technologies and can set the foundation for innovative services and applications. They are particularly relevant in areas without existing telecommunications infrastructure or where the existing infrastructure is insufficient to meet current and future demands.
The official release dates of Wi-Fi 4, Wi-Fi 5, Wi-Fi 6, and Wi-Fi 7 (as per the IEEE standardization and Wi-Fi Alliance naming conventions) are as follows:
- Wi-Fi 4 (IEEE 802.11n):
- Standard Finalized: October 2009
- Wi-Fi 4 is the designation given to the IEEE 802.11n standard, which significantly improved upon previous Wi-Fi standards by introducing technologies like MIMO and increased data rates.
- Wi-Fi 5 (IEEE 802.11ac):
- Standard Finalized: January 2014 (Wave 1), December 2013 (Wave 2)
- Wi-Fi 5 refers to the IEEE 802.11ac standard, which enhanced Wi-Fi performance further by introducing features like wider channel bandwidth and support for additional spatial streams.
- Wi-Fi 6 (IEEE 802.11ax):
- Standard Finalized: September 2019
- Wi-Fi 6, known technically as IEEE 802.11ax, brought significant advancements in efficiency, especially in crowded environments, and introduced technologies like OFDMA and Target Wake Time (TWT).
- Wi-Fi 7 (IEEE 802.11be):
- Expected Finalization: As of my last update in April 2023, Wi-Fi 7 (IEEE 802.11be) was still in development, with finalization expected around 2024.
- Wi-Fi 7 is expected to offer further improvements in terms of data rates, latency, and efficiency, continuing the evolution of Wi-Fi technology.
These release dates mark important milestones in the development of Wi-Fi technology, with each new generation bringing enhancements that have enabled faster speeds, greater capacity, and more efficient network performance.
Roaming service refers to the ability of a cell phone or mobile device user to automatically make and receive voice calls, send and receive data, or access other services when traveling outside the geographical coverage area of their home network, by using a visited network. This service is essential for maintaining connectivity when users are in areas not served by their carrier’s regular network. Key aspects of roaming services include:
- Types of Roaming:
- Domestic Roaming: Occurs when a user connects to another operator’s network within their home country.
- International Roaming: Involves using a mobile device on a foreign operator’s network while traveling abroad.
- Roaming Agreements: Mobile operators form agreements with other operators to provide roaming services to their subscribers. These agreements cover aspects like service standards, pricing, and data exchange.
- Seamless Connectivity: Roaming is designed to provide seamless service, with users able to use their mobile phones for calls, text messages, and data services just as they do at home.
- Charges and Tariffs: Roaming often incurs additional charges, which can vary significantly depending on the operators involved and the user’s service plan. International roaming, in particular, can be expensive.
- SIM Card and Network Compatibility: Effective roaming depends on the compatibility of the user’s mobile device and SIM card with the visited network, particularly in terms of supported frequency bands and network technology.
- Roaming Partners and Coverage: Mobile operators typically publish lists of their roaming partners and the countries where roaming services are available.
- Regulatory Aspects: In some regions, like the European Union, regulations have been put in place to control roaming charges and protect consumers from high fees.
- Data Roaming: This allows users to access the internet and use data-driven services. Data roaming can be particularly costly, and users often have the option to disable it.
Roaming services are a critical aspect of global telecommunications, enabling users to stay connected while traveling outside their home network’s coverage area.
GSM (Global System for Mobile Communications) is a standard developed by the European Telecommunications Standards Institute (ETSI) to describe the protocols for second-generation (2G) digital cellular networks used by mobile devices such as phones and tablets. Introduced in the 1990s, GSM was a major leap in mobile communication technology. Key aspects of GSM include:
- Digital Communication: GSM marked the transition from analog first-generation (1G) networks to digital, significantly improving voice quality, security, and capacity.
- Global Standard: As its name suggests, GSM became a global standard for mobile communication, facilitating international roaming and compatibility.
- Network Components: GSM networks consist of key subsystems like the Base Station Subsystem (BSS), Network and Switching Subsystem (NSS), and the Operations and Support Subsystem (OSS).
- SIM Cards: GSM introduced the use of SIM (Subscriber Identity Module) cards, which store subscriber data and facilitate mobile device identification and authentication on the network.
- Data Services: Besides voice communication, GSM supports data services such as SMS (Short Message Service) and later, GPRS (General Packet Radio Services) for basic internet connectivity.
- Encryption and Security: GSM networks employ encryption to secure voice and data communication, enhancing privacy and security.
- Frequency Bands: GSM operates in multiple frequency bands, like 900 MHz and 1800 MHz in Europe and 850 MHz and 1900 MHz in the Americas, catering to different regional requirements.
GSM set the foundation for modern mobile communication and led to the development of more advanced technologies like 3G (UMTS) and 4G (LTE).
Organizations (5)
Mobile operators, also known as mobile network operators (MNOs), are companies that provide wireless voice and data communication services to mobile device users. They are an essential part of the telecommunications industry. Key aspects of mobile operators include:
- Network Infrastructure: Mobile operators own or control access to the network infrastructure necessary to provide services to mobile phone subscribers. This includes cell towers, networking equipment, and back-end systems.
- Service Provisioning: They offer various services such as voice calls, text messaging (SMS), multimedia messaging (MMS), and internet access. With advancements in technology, services have expanded to include mobile broadband, streaming, and more.
- Spectrum Licensing: Mobile operators typically acquire licenses to operate in specific frequency bands from government regulatory bodies. This spectrum is crucial for transmitting and receiving wireless signals.
- Technology Adoption: They are responsible for upgrading their networks to support newer technologies (e.g., transitioning from 3G to 4G LTE, and now to 5G), enhancing speed, capacity, and service quality.
- Subscriber Management: Mobile operators manage customer relationships, including billing, customer service, and offering various plans and packages to cater to different user needs.
- Regulatory Compliance: They must comply with the regulations and policies set by telecommunications regulatory authorities, which may include aspects like service quality, fair competition, and emergency services.
- Roaming Services: Mobile operators often establish agreements with operators in other regions or countries to provide service to their subscribers when they are outside their home network (roaming).
- Value-Added Services: Besides basic communication services, many operators offer additional services like music streaming, video content, cloud storage, and digital payments.
Mobile operators play a crucial role in connecting people and devices, driving innovation in the telecommunications sector, and facilitating the growth and adoption of new mobile technologies.
The European Telecommunications Standards Institute (ETSI) is an independent, non-profit standardization organization for the telecommunications industry in Europe, with a worldwide influence. ETSI plays a significant role in developing global standards for Information and Communication Technologies (ICT), including fixed, mobile, radio, converged, broadcast, and internet technologies. Key aspects of ETSI include:
- Standard Development: ETSI is responsible for creating internationally-applicable standards across a wide range of telecommunications and ICT services and technologies.
- Global Influence: While ETSI is focused on Europe, its standards are often adopted worldwide. The GSM standard developed by ETSI is a prime example of its global impact.
- Membership: ETSI’s members include manufacturers, network operators, service providers, research bodies, and national administrations from across the globe.
- Collaboration with Other Bodies: ETSI collaborates with other standardization organizations like ITU (International Telecommunication Union) and 3GPP (3rd Generation Partnership Project) to ensure global alignment and interoperability of standards.
- Innovation and Technology Development: ETSI is involved in emerging and future-oriented technologies, playing a key role in areas like 5G, IoT (Internet of Things), and cybersecurity.
- Standards for New Technologies: ETSI has been instrumental in developing standards for various new technologies, including those related to network functions virtualization (NFV), software-defined networking (SDN), and more.
ETSI’s work ensures compatibility and interoperability of systems, which is vital for the global telecommunications industry, fostering innovation and facilitating seamless communication and connectivity.
The GSMA (Global System for Mobile Communications Association) is an industry organization that represents the interests of mobile network operators worldwide. Established in 1987, it plays a central role in shaping the future of mobile communications and the wider mobile ecosystem. Here are some key aspects of the GSMA:
- Membership and Representation: The GSMA has a large and diverse membership that includes nearly 800 mobile operators and more than 300 companies in the broader mobile ecosystem, including handset manufacturers, software companies, equipment providers, and internet companies.
- Standards and Policies: One of its main roles is to develop and promote mobile industry standards and policies. The GSMA works closely with standardization bodies, governments, and other organizations to foster a collaborative environment for standard development.
- Mobile World Congress: The GSMA is perhaps best known for organizing the Mobile World Congress (MWC) events, which are among the largest annual exhibitions and conferences in the mobile industry, held in different locations around the world.
- Advocacy and Research: The organization advocates on behalf of its members on a range of issues, from regulatory and public policy to technology and health matters. It also conducts research and publishes reports on various aspects of the mobile industry.
- Sustainability and Social Impact: The GSMA is involved in initiatives that use mobile technology for positive social and economic impact. This includes efforts in areas such as environmental sustainability, digital inclusion, and emergency response.
- 5G Development: The GSMA plays a significant role in the development and adoption of 5G technology, collaborating with industry stakeholders to establish standards and ensure a smooth rollout of 5G networks.
In summary, the GSMA is a key global organization in the mobile communications industry, facilitating collaboration, innovation, and strategic development to benefit mobile operators and the wider mobile ecosystem.
The Federal Communications Commission (FCC) is an independent agency of the United States government created by statute (47 U.S.C. § 151 and 47 U.S.C. § 154) to regulate interstate communications by radio, television, wire, satellite, and cable. It plays a key role in managing communication technologies and services in the U.S. Key aspects of the FCC include:
- Regulation and Oversight: The FCC regulates all non-federal government use of the radio spectrum (including radio and television broadcasting), all interstate telecommunications (wire, satellite, and cable), and international communications that originate or terminate in the United States.
- Licensing: It is responsible for licensing radio and television stations, and ensuring compliance with the relevant regulations.
- Promoting Competition: The FCC works to promote competition, innovation, and investment in broadband services and facilities.
- Spectrum Management: One of its crucial roles is managing the nation’s airwaves, including spectrum allocation and assignment for various uses.
- Consumer Protection: The agency also enforces laws to protect consumers against fraud, unfair practices, and monopolistic behavior in the communications realm.
- Policy Making: The FCC develops policy concerning issues such as media ownership, net neutrality, privacy, and others that impact the nation’s communications.
- Emergency Communications: It plays a significant role in ensuring the reliability and security of critical communications infrastructure, particularly during emergencies.
- Digital Transition: The FCC has been instrumental in overseeing the transition from analog to digital broadcasting and the development and deployment of new communication technologies like 5G.
The FCC’s actions are watched closely by various stakeholders due to their far-reaching impact on how Americans communicate and access information.
The International Telecommunication Union (ITU) is a specialized agency of the United Nations responsible for issues that concern information and communication technologies. Established in 1865, originally as the International Telegraph Union, the ITU is one of the oldest international organizations. It plays a pivotal role in facilitating global communications and technology standards. Key aspects of the ITU include:
- Standardization: The ITU is responsible for developing international standards (ITU-T Recommendations) that facilitate seamless global telecommunications and ensure interoperable and efficient communication systems.
- Radio Spectrum Allocation: The ITU coordinates the global use of the radio spectrum (ITU-R Recommendations) and satellite orbits, ensuring non-interference and efficient use of these resources.
- Improving Access to ICTs: The organization works to improve access to information and communication technologies (ICTs) in underserved communities worldwide, promoting sustainable development.
- Regulatory Framework and Policies: ITU assists in developing regulatory frameworks and offers policy advice to ensure fair and equitable access to ICT services.
- Telecommunication Development: The ITU-D sector focuses on fostering international cooperation and solidarity in the delivery of technical assistance and the implementation of telecommunication/ICT projects in developing countries.
- Global Conferences and Exhibitions: ITU organizes the World Radiocommunication Conference (WRC), World Telecommunication Standardization Assembly (WTSA), and other significant events that shape the future of ICTs.
- Membership: ITU’s membership includes 193 Member States as well as over 800 private-sector entities, academic institutions, and international and regional organizations.
The ITU plays a crucial role in shaping global telecommunications’s technological and regulatory landscape, making it a cornerstone entity in modern communication and information exchange.
WIreless Technologies (20)
The evolution of Radio Access Network (RAN) solutions from 2G to 5G represents a journey of significant technological advancements, each generation introducing new capabilities and features. Here is an overview of how RAN solutions have evolved:
- 2G (GSM) RAN:
- Introduced in the 1990s, 2G was the first generation of digital cellular technology.
- It primarily focused on voice services and simple data transmission using technologies like GSM (Global System for Mobile Communications).
- 2G RANs utilized narrowband TDMA (Time Division Multiple Access).
- 3G RAN:
- Launched in the early 2000s, 3G brought higher data rates, enabling mobile internet access and improved voice call quality.
- Technologies like UMTS (Universal Mobile Telecommunications System) and later HSPA (High-Speed Packet Access) were used.
- 3G RANs used wideband CDMA (Code Division Multiple Access) for more efficient spectrum utilization.
- 4G (LTE) RAN:
- 4G, introduced in the late 2000s, marked a significant leap with LTE (Long-Term Evolution) technology, offering high-speed mobile broadband.
- LTE RANs provided much higher data rates, lower latency, and improved capacity compared to 3G.
- The focus shifted towards all-IP (Internet Protocol) based networks, enabling seamless internet and multimedia services.
- 5G RAN:
- 5G, rolling out since 2019, introduces even higher data rates, ultra-low latency, and massive network capacity.
- It supports advanced applications like IoT, augmented reality, and autonomous vehicles.
- 5G RANs utilize technologies like Massive MIMO (Multiple Input Multiple Output), beamforming, and network slicing.
- They operate across a broader range of frequencies, including sub-6 GHz and mmWave bands.
Each generation’s RAN has been characterized by advancements in data rate, efficiency, and the types of services it could support. From basic voice and text in 2G to multimedia and high-speed data in 4G, and now to a fully connected world with 5G, the RAN technology has continuously evolved to meet the growing demands of connectivity and innovation.
Radio Access Network (RAN) solutions are integral components of mobile telecommunications networks. They encompass the technology and infrastructure responsible for connecting mobile devices to the core network and managing wireless communication. Key aspects of RAN solutions include:
- Connection Handling: RAN solutions handle all radio connections between user devices (like smartphones and tablets) and the network, facilitating communication with the core network.
- Base Stations and Antennas: They comprise base stations (also known as cell sites) and antennas that cover specific geographic areas, known as cells. These base stations manage radio communication with devices in their coverage area.
- Types of RAN:
- Traditional RAN: In traditional RAN setups, hardware and software are typically proprietary and supplied by a single vendor. The network elements are closely integrated.
- Open RAN: Open RAN architectures promote open interfaces and interoperability between different vendors’ equipment, allowing more flexibility and vendor diversity.
- Technology Evolution: RAN solutions have evolved from 2G to 5G, with each generation bringing advancements in speed, capacity, and efficiency. Current developments focus on 5G RAN, offering high-speed data transmission and low latency.
- Virtualization and Centralization: Modern RAN solutions are moving towards virtualization and centralization, where traditional hardware elements are replaced with software-defined solutions that can be centrally managed.
- Cloud RAN (C-RAN): An emerging approach where RAN functionalities are hosted in cloud data centers, leading to more efficient resource utilization and better network management.
- Support for Diverse Applications: RAN solutions support a wide range of applications, from voice calls and texting to high-speed internet access, streaming, and IoT connectivity.
RAN solutions are crucial for the functioning of mobile networks, serving as the link between end-users and the broader network infrastructure, and are continually evolving to meet the demands of new technologies and applications.
The frequency bands used in global telecommunications are varied and designated for specific purposes, including mobile communication, broadcasting, satellite communication, and more. Here’s an overview of some key frequency bands used in telecom:
- Low Frequency (LF) Bands (30 kHz to 300 kHz):
- Primarily used for AM radio broadcasting, maritime communication, and navigation.
- Medium Frequency (MF) Bands (300 kHz to 3 MHz):
- Used for AM radio broadcasting and aviation communication.
- High Frequency (HF) Bands (3 MHz to 30 MHz):
- Utilized for shortwave radio broadcasting, amateur radio, and maritime communication.
- Very High Frequency (VHF) Bands (30 MHz to 300 MHz):
- Include FM radio broadcasting (88 MHz to 108 MHz) and VHF TV broadcasting.
- Used in aviation and maritime communication, and two-way radios.
- Ultra High Frequency (UHF) Bands (300 MHz to 3 GHz):
- Cover TV broadcasting and mobile communication (LTE, GSM).
- Include the 2.4 GHz band used for Wi-Fi and Bluetooth.
- Super High Frequency (SHF) Bands (3 GHz to 30 GHz):
- Encompass parts of the spectrum used for newer 4G and 5G cellular networks.
- Include bands used for satellite communication and radar systems.
- Extremely High Frequency (EHF) Bands (30 GHz to 300 GHz):
- Used in high-capacity wireless communication, millimeter-wave radar, and scientific research.
- 5G networks utilize some of these higher frequencies (e.g., around 28 GHz and 39 GHz) for mmWave communication.
- Cellular Frequency Bands:
- GSM Bands: 900 MHz and 1800 MHz in most parts of the world, 850 MHz and 1900 MHz in the Americas.
- 3G/UMTS Bands: 2100 MHz (Band 1) is the most widely used globally.
- 4G/LTE Bands: Numerous bands including 700 MHz, 800 MHz, 1800 MHz, 2600 MHz, and others.
- 5G Bands: Ranging from sub-1 GHz low bands to mid-band (3.5 GHz) and high-band mmWave frequencies.
The allocation of these bands can vary by region, and they are regulated by international organizations like the International Telecommunication Union (ITU) and national regulatory bodies such as the FCC in the United States or Ofcom in the United Kingdom.
A Radio Intelligent Controller (RIC) is a key component in modern wireless network architectures, particularly in Open Radio Access Networks (Open RAN or O-RAN). The RIC plays a critical role in optimizing and managing radio network functions through advanced algorithms and machine learning. Key aspects of a Radio Intelligent Controller include:
- Network Optimization: The RIC uses real-time analytics to optimize network performance, including managing resources, balancing loads, and enhancing connectivity.
- Automation and Intelligence: By incorporating artificial intelligence and machine learning, the RIC automates many network operations, improving efficiency and reducing the need for manual intervention.
- Open RAN Integration: In the context of Open RAN, the RIC is crucial for enabling interoperability and flexibility, allowing components from different vendors to work seamlessly together.
- Two Types of RICs:
- Near-Real-Time RIC: Focuses on optimizing network performance in a timescale of milliseconds to seconds. It manages functions like handovers, beamforming, and load balancing.
- Non-Real-Time RIC: Operates on a longer timescale (seconds to minutes) and is involved in broader network management functions like policy control, network slicing, and predictive analysis.
- Standardization and Open Interfaces: The development of RICs is guided by standardization bodies like the O-RAN Alliance, which promotes open interfaces and standardized software to foster innovation and vendor diversity.
- Enhanced User Experience: By optimizing network performance, RICs contribute to an enhanced user experience, offering better connectivity, reduced latency, and more reliable service.
- Scalability and Flexibility: RICs enable networks to scale more efficiently and adapt to changing demands, supporting the rollout of new services and technologies like 5G.
The RIC represents a significant evolution in radio network management, bringing intelligence and flexibility to the forefront of wireless network operations.
Adaptive Data Rate (ADR) is a feature in some wireless communication protocols, notably in LoRaWAN (Long Range Wide Area Network), which is part of the broader category of Low Power Wide Area Networks (LPWAN). ADR optimizes the data transmission rate, power consumption, and airtime of devices based on network conditions, device power capacity, and the quality of the radio link. Here’s how ADR functions and its importance:
- Optimization of Data Rate: ADR dynamically adjusts the data rate at which a device transmits. This is done by changing the spreading factor, bandwidth, and coding rate. Higher data rates can be used when the device is near a gateway, while lower data rates are used as the device moves further away.
- Power Efficiency: By adjusting the data rate, ADR also helps in conserving the battery life of devices. Devices that are closer to a gateway and can transmit at higher data rates will use less power, thus preserving battery life.
- Network Capacity Management: ADR helps in managing the capacity of the network. By ensuring that devices use the optimal data rate, it reduces the time on air for each transmission. This efficiency is crucial in LPWANs as it increases the overall capacity of the network to handle more devices.
- Adaptation to Changing Conditions: ADR responds to changing environmental conditions or changes in the location of the device. If a device’s transmissions start failing, ADR can lower the data rate to increase the chance of successful transmission.
- Manual Override: In some systems, ADR can be manually overridden. This is useful in scenarios where the network administrator knows the environment and can set the data rate to a fixed value for optimal performance.
- Use in LoRaWAN: In LoRaWAN, ADR is a critical feature, especially considering the varying distances between end devices and gateways and the need for long battery life in IoT applications.
- Limitations: ADR is not always suitable for devices that are mobile or experience rapidly changing RF conditions, as it may not react quickly enough to these changes.
In summary, Adaptive Data Rate is a key feature in wireless communication protocols like LoRaWAN, enhancing network efficiency, power consumption, and overall performance of the connected devices. It is particularly important in scenarios where devices must operate over extended periods on limited power sources, such as in many IoT applications.
GSM (Global System for Mobile Communications) is a standard developed by the European Telecommunications Standards Institute (ETSI) to describe the protocols for second-generation (2G) digital cellular networks used by mobile devices such as phones and tablets. Introduced in the 1990s, GSM was a major leap in mobile communication technology. Key aspects of GSM include:
- Digital Communication: GSM marked the transition from analog first-generation (1G) networks to digital, significantly improving voice quality, security, and capacity.
- Global Standard: As its name suggests, GSM became a global standard for mobile communication, facilitating international roaming and compatibility.
- Network Components: GSM networks consist of key subsystems like the Base Station Subsystem (BSS), Network and Switching Subsystem (NSS), and the Operations and Support Subsystem (OSS).
- SIM Cards: GSM introduced the use of SIM (Subscriber Identity Module) cards, which store subscriber data and facilitate mobile device identification and authentication on the network.
- Data Services: Besides voice communication, GSM supports data services such as SMS (Short Message Service) and later, GPRS (General Packet Radio Services) for basic internet connectivity.
- Encryption and Security: GSM networks employ encryption to secure voice and data communication, enhancing privacy and security.
- Frequency Bands: GSM operates in multiple frequency bands, like 900 MHz and 1800 MHz in Europe and 850 MHz and 1900 MHz in the Americas, catering to different regional requirements.
GSM set the foundation for modern mobile communication and led to the development of more advanced technologies like 3G (UMTS) and 4G (LTE).
Wi-Fi HaLow, designated as 802.11ah, is a wireless networking protocol developed by the Wi-Fi Alliance. It’s a part of the IEEE 802.11 set of WLAN standards, but it differs significantly from most of its predecessors. Here are some key aspects of Wi-Fi HaLow:
- Frequency Band: Wi-Fi HaLow operates in the sub-1 GHz spectrum, specifically in the 900 MHz band. This is a lower frequency compared to the 2.4 GHz and 5 GHz bands used by most Wi-Fi technologies. The lower frequency allows for better range and material penetration.
- Range and Coverage: One of the most significant benefits of Wi-Fi HaLow is its extended range. It can cover roughly double the distance of conventional Wi-Fi, making it ideal for reaching into areas that were previously difficult to cover.
- Penetration: The lower frequency also allows for better penetration through obstacles like walls and floors, making Wi-Fi HaLow more reliable in challenging environments.
- Power Efficiency: Wi-Fi HaLow is designed to be more power-efficient, which is crucial for Internet of Things (IoT) devices that often run on batteries. This efficiency extends the battery life of connected devices.
- IoT Applications: Due to its range, penetration, and power efficiency, Wi-Fi HaLow is particularly well-suited for IoT applications, especially in scenarios where devices need to be connected over larger areas or in challenging environments, like smart homes, agricultural settings, industrial sites, and smart cities.
- Device Connectivity: It supports a larger number of connected devices over a single access point compared to traditional Wi-Fi, which is beneficial for IoT environments where many devices need to be connected.
- Security and IP Support: Wi-Fi HaLow retains the high levels of security and native IP support that are characteristic of traditional Wi-Fi standards.
In summary, Wi-Fi HaLow extends the benefits of Wi-Fi to IoT applications, offering solutions to the unique challenges posed by the need for long-range, low-power, high-penetration wireless connectivity. It’s particularly relevant as the number of IoT devices continues to grow, requiring new solutions for connectivity.
LoRaWAN (Long Range Wide Area Network) is a protocol for low-power wide-area networks (LPWANs), designed to wirelessly connect battery-operated ‘things’ to the internet in regional, national, or global networks. It’s particularly useful for the Internet of Things (IoT) applications. Here are some key characteristics and aspects of LoRaWAN:
- Long Range Communication: LoRaWAN is known for its long-range capabilities, often reaching several kilometers in rural areas and penetrating dense urban or indoor environments.
- Low Power Consumption: Devices using LoRaWAN are designed to be power-efficient, which is critical for IoT applications where devices often run on batteries and need to operate for extended periods without maintenance.
- Secure Communication: LoRaWAN includes end-to-end encryption, ensuring secure data transmission, which is crucial in many IoT applications.
- Low Bandwidth: LoRaWAN is optimized for low data rate applications. It’s not suitable for large amounts of data or high-speed communication but is ideal for applications that only need to send small amounts of data over long intervals.
- Star-of-Stars Network Topology: In LoRaWAN networks, gateways relay messages between end-devices and a central network server. The gateways are connected to the network server via standard IP connections, while end-devices use single-hop wireless communication to one or many gateways.
- Adaptive Data Rate (ADR): LoRaWAN can optimize data rates and RF output to balance power consumption, airtime, and network capacity.
- Applications: It’s used in a variety of applications, including smart meters, smart agriculture, smart cities, and environmental monitoring.
- Network Architecture: The architecture is typically laid out in a hierarchical topology to enhance scalability and battery life for end-devices.
- License-Free Frequency Band: LoRaWAN operates in license-free bands such as the industrial, scientific, and medical (ISM) radio bands.
LoRaWAN is an essential technology for IoT ecosystems, especially in scenarios where devices need to communicate over long distances, consume minimal power, and send small amounts of data.
Low Power Wide Area Networks (LPWAN) are a type of wireless telecommunication network designed to allow long-range communications at a low bit rate among connected devices, typically used for M2M (Machine to Machine) and IoT (Internet of Things) applications. Key characteristics and advantages of LPWAN include:
- Long Range: LPWAN technologies are designed to provide wide-area coverage, often covering a radius of several kilometers, even in challenging environments such as urban or industrial areas.
- Low Power Consumption: Devices connected via LPWAN are optimized for low power consumption, which allows them to operate for years on a small battery. This is crucial for IoT applications where devices are often deployed in locations where regular maintenance or battery replacement is not feasible.
- Low Data Rate: LPWAN is optimized for transmissions that require a low data rate. It’s ideal for applications that only need to send small amounts of data intermittently, rather than streaming large quantities of data continuously.
- Cost-Effectiveness: The infrastructure and device costs associated with LPWAN are generally lower compared to other types of wireless networks. This makes LPWAN a practical choice for a wide range of IoT applications.
- Applications: LPWAN is used in a variety of applications, including smart meters, smart agriculture, asset tracking, and environmental monitoring.
- Examples of LPWAN Technologies: Some of the well-known LPWAN technologies include LoRaWAN (Long Range Wide Area Network), NB-IoT (Narrowband IoT), and Sigfox.
In summary, LPWANs play a crucial role in the growth of IoT by connecting devices over long distances with minimal power consumption and lower costs, making it feasible to deploy large networks of sensors and devices.
MIMO (Multiple Input Multiple Output) is a wireless technology used in communication systems, particularly in modern Wi-Fi and cellular networks like LTE and 5G. It involves the use of multiple antennas at both the transmitter and receiver to improve communication performance. Key aspects of MIMO include:
- Increased Data Throughput: By using multiple antennas, MIMO can transmit more data simultaneously compared to systems with a single antenna, significantly increasing the network’s data throughput.
- Spatial Multiplexing: This technique, used in MIMO systems, transmits different data streams simultaneously over the same frequency band but through different spatial paths. It effectively multiplies the capacity of the radio channel.
- Diversity Gain: MIMO can provide diversity gain by transmitting the same data across different antennas, reducing the likelihood of data loss due to fading or interference.
- Improved Signal Quality: MIMO systems can improve signal quality and reduce error rates by combining multiple received signals, which have traveled through different paths and thus experienced different levels of fading and interference.
- Beamforming: Advanced MIMO systems use beamforming to direct the signal towards the intended receiver, enhancing the signal strength and reducing interference to and from other devices.
- Types of MIMO:
- SU-MIMO (Single-User MIMO): Involves one transmitter and one receiver, each with multiple antennas.
- MU-MIMO (Multi-User MIMO): Allows communication with multiple users simultaneously, each with one or more antennas.
- Applications: MIMO technology is a foundational element in modern wireless communication standards, including Wi-Fi (802.11n, ac, ax), LTE, and 5G networks.
MIMO technology represents a significant advancement in wireless communications, enabling more efficient and reliable transmission of data, and is essential for achieving the high-speed and high-capacity requirements of current and future wireless networks.
Open RAN (Open Radio Access Network) is an initiative to create more open and interoperable wireless network architectures. It represents a shift from traditional RAN solutions, which often involve proprietary, integrated systems from a single vendor, to a more modular and flexible approach. Key aspects of Open RAN include:
- Open Interfaces: Open RAN emphasizes the use of standardized, open interfaces between various components of the radio access network. This allows for interoperability between different vendors’ equipment.
- Decoupling Hardware and Software: It enables the decoupling of hardware and software functionalities in the network, allowing operators to mix and match hardware and software from different suppliers.
- Vendor Diversity and Innovation: By promoting open standards and interfaces, Open RAN encourages more vendors to participate in the ecosystem, fostering innovation and potentially reducing costs.
- Virtualization and Software-Defined Networking: Open RAN leverages virtualization technologies and software-defined networking (SDN) principles, leading to more flexible and scalable networks.
- Increased Efficiency and Agility: Networks based on Open RAN can adapt more quickly to changing demands and technologies, improving efficiency and service quality.
- Support for 5G and Beyond: Open RAN is seen as a key enabler for the rollout of 5G networks, offering the agility and scalability needed to support 5G’s diverse use cases.
- Organizations and Alliances: Several industry alliances and organizations, such as the O-RAN Alliance and the Telecom Infra Project (TIP), are driving the development and adoption of Open RAN standards.
Open RAN represents a transformative approach in the deployment and operation of mobile networks, promising to enhance competition, innovation, and flexibility in the telecom industry.
Quadrature Amplitude Modulation (QAM) is a modulation technique used in various forms of communication systems, including digital television and wireless communications. It combines two amplitude-modulated signals into a single channel, thereby increasing the bandwidth efficiency. Here’s a more detailed look at QAM:
- Combining Amplitude and Phase Modulation: QAM works by varying both the amplitude and the phase of a carrier signal. Essentially, it’s a blend of both amplitude modulation (AM) and phase modulation (PM).
- Constellation Diagram: In QAM, data points represented in a modulation are often visualized using a constellation diagram, which plots the amplitude and phase variations as points on a two-dimensional graph. Each point on the diagram represents a different symbol.
- Increased Data Rates: By varying both amplitude and phase, QAM can transmit more data per symbol compared to using either modulation technique alone. This makes it more bandwidth-efficient and enables higher data transmission rates.
- Applications in Digital Transmission: QAM is widely used in digital radio and television broadcasting, cable TV systems, and in some wireless communication systems like Wi-Fi and cellular networks.
- Variants of QAM: There are several variants of QAM, like 16-QAM, 64-QAM, 256-QAM, and others. The number denotes how many different symbols can be represented; for instance, 256-QAM can represent 256 different symbols. Higher QAM levels can transmit more bits per symbol, but they also require a higher signal-to-noise ratio to avoid errors.
- Adaptive QAM: In some communication systems, QAM can be adaptively changed depending on the channel conditions. For example, a system might use a higher level of QAM when signal conditions are good and a lower level when they are less favorable to maintain the quality of the transmission.
- Challenges with Higher QAM Levels: As the level of QAM increases, the spacing between constellation points becomes tighter, making the system more susceptible to noise and errors. Hence, higher QAM levels require better quality transmission channels.
In summary, QAM is a fundamental modulation technique that enables efficient use of available bandwidth by combining amplitude and phase modulation, widely used in modern digital communication systems.
Spectrum licensing refers to the regulatory process whereby national governments or regulatory bodies authorize the use of specific parts of the radio frequency spectrum by individuals, companies, or organizations. This process is crucial for managing the radio spectrum, which is a finite resource. Key aspects of spectrum licensing include:
- Regulatory Authority Involvement: Spectrum licensing is typically overseen by a national regulatory authority, such as the Federal Communications Commission (FCC) in the United States or Ofcom in the United Kingdom.
- Allocation and Assignment: The process involves allocating frequency bands for specific uses (such as mobile communication, broadcasting, or satellite transmission) and assigning specific frequencies or bands to licensees.
- License Types: There are various types of spectrum licenses, including exclusive use licenses, shared use licenses, and unlicensed spectrum allocations (like the bands used for Wi-Fi).
- Auctioning Spectrum: Many countries use auctions to allocate spectrum licenses, allowing companies to bid on the rights to use certain frequency bands. This method is often used for commercial purposes like mobile networks.
- Licensing Fees: Licensees typically pay a fee for spectrum use rights. Fees can vary based on the spectrum band, the geographic coverage of the license, and the duration of the license.
- Conditions and Regulations: Spectrum licenses come with conditions and regulations to ensure efficient and non-interfering use of the spectrum, including technical specifications, usage limitations, and compliance with international agreements.
- Spectrum Management: Effective spectrum licensing is a critical aspect of spectrum management, ensuring that this valuable resource is used efficiently and in a way that minimizes interference between different users.
- Economic and Strategic Importance: Spectrum licensing is not only a regulatory process but also of significant economic and strategic importance, influencing the development and deployment of wireless communication technologies.
Spectrum licensing is a key tool in the management of radio frequencies, balancing the need for efficient use of the spectrum, technological innovation, and economic considerations.
Spectrum Reallocation Strategy refers to the process of reassigning and repurposing frequency bands for different uses, typically within the context of wireless communications. This strategy is crucial in managing the finite resource of the radio spectrum, especially with the increasing demand for wireless services. Key aspects of Spectrum Reallocation Strategy include:
- Addressing Spectrum Scarcity: With the growing number of wireless devices and services, such as mobile phones, IoT devices, and broadband services, the demand for radio spectrum has significantly increased, leading to the need for efficient spectrum management.
- Reallocating for New Technologies: As new technologies like 5G emerge, reallocating spectrum bands to accommodate these technologies becomes necessary to ensure they have the necessary bandwidth to operate effectively.
- Balancing Interests: The process involves balancing the needs and interests of various stakeholders, including government agencies, private sector companies, and the public.
- Regulatory Decisions: National and international regulatory bodies, such as the Federal Communications Commission (FCC) in the U.S. or the International Telecommunication Union (ITU) globally, play a key role in making decisions about spectrum reallocation.
- Auctioning Spectrum: Often, reallocated spectrum is auctioned off to the highest bidder, providing a transparent and market-driven mechanism for allocation.
- Minimizing Disruption: Careful planning is required to minimize disruption to existing services and users in bands that are being reallocated.
- Economic Implications: Spectrum reallocation can have significant economic implications, both in terms of the revenue generated from spectrum auctions and the economic benefits of new technologies and services that use the reallocated spectrum.
Spectrum Reallocation Strategy is a critical aspect of modern telecommunications policy, ensuring that this valuable resource is used effectively to meet current and future needs.
A “License-Free Frequency Band” refers to parts of the radio spectrum that can be used without the need to acquire a license from regulatory authorities. These bands are open for public use under certain regulations and guidelines set by the governing bodies, like the Federal Communications Commission (FCC) in the United States or similar organizations in other countries. Key features and implications of these bands include:
- Open Access: Individuals and companies can operate devices in these bands without needing to obtain a license, which lowers the barriers to entry for developing and deploying wireless technologies.
- Regulatory Guidelines: Although no license is required, there are still regulations that govern the use of these bands. These typically include limits on transmission power, requirements for equipment to tolerate interference, and rules to minimize the risk of devices interfering with each other.
- Common Uses: License-free bands are commonly used for consumer wireless devices like Wi-Fi routers (in the 2.4 GHz and 5 GHz bands), Bluetooth devices, cordless telephones, and other short-range communication devices. They’re also used for industrial, scientific, and medical (ISM) applications.
- Popular License-Free Bands: The most well-known license-free bands are the ISM bands, which include frequencies around 900 MHz, 2.4 GHz, and 5 GHz. These bands are widely used for a variety of wireless communication technologies.
- Advantages: The primary advantage of using license-free bands is the reduced cost and complexity associated with bringing a wireless product to market. There’s no need to bid for spectrum rights or pay licensing fees.
- Challenges: A major challenge in these bands is the potential for interference, as many different devices and technologies may be operating in the same frequency range. This can impact the performance and reliability of wireless communications.
- Global Variation: The availability and specific regulations of license-free bands can vary from one country to another, so manufacturers need to ensure their devices comply with the regulations in each market where they are sold.
In summary, license-free frequency bands are crucial for a wide range of wireless technologies, especially for consumer and small-scale industrial applications. They enable easier access to the radio spectrum but come with the responsibility of adhering to regulations and managing interference.
Wi-Fi 4, officially known as IEEE 802.11n, is the fourth generation of Wi-Fi standards and was a significant advancement over the previous Wi-Fi standards, particularly IEEE 802.11g. Introduced in 2009, Wi-Fi 4 brought several key improvements to wireless networking:
- Increased Speed: Wi-Fi 4 offered higher maximum data rates, up to 600 Mbps under ideal conditions, which was a substantial improvement over the 54 Mbps maximum of its predecessor.
- MIMO Technology: Wi-Fi 4 introduced Multiple Input Multiple Output (MIMO) technology. This allowed the use of multiple antennas for both transmission and reception, enhancing data throughput and signal range.
- Dual-Band Operation: Wi-Fi 4 could operate on both the 2.4 GHz and 5 GHz bands, giving users the flexibility to choose the band with less interference and better performance.
- Wider Channel Bandwidth: It supported channel bandwidths of up to 40 MHz, wider than the 20 MHz channels of previous standards. This allowed for more data to be transmitted simultaneously.
- Improved Range and Reliability: The range and reliability of Wi-Fi connections were significantly improved, offering better performance at greater distances and in environments with physical obstructions.
- Backward Compatibility: Wi-Fi 4 was backward compatible with earlier Wi-Fi standards, ensuring that devices supporting older standards could still connect to Wi-Fi 4 networks.
Wi-Fi 4 played a crucial role in advancing wireless networking technology, facilitating faster speeds, increased range, and better overall performance, paving the way for the development of subsequent Wi-Fi generations.
Wi-Fi 5, known technically as IEEE 802.11ac, is the fifth generation of Wi-Fi standards. It was a significant improvement over its predecessor, Wi-Fi 4 (802.11n), and brought several advancements:
- Higher Data Rates: Wi-Fi 5 offers greater maximum data rates than Wi-Fi 4, primarily due to more efficient data encoding. This results in faster speeds for users.
- Dual-Band Operation: Unlike Wi-Fi 4, which primarily operates on the 2.4 GHz band, Wi-Fi 5 operates on both 2.4 GHz and 5 GHz bands. The 5 GHz band offers less interference and higher speeds.
- Wider Channel Bandwidth: Wi-Fi 5 supports wider channel bandwidths of up to 160 MHz (compared to the maximum of 40 MHz in Wi-Fi 4), allowing more data to be transmitted simultaneously.
- MU-MIMO (Multi-User, Multiple Input, Multiple Output): This technology enables a Wi-Fi router to communicate with multiple devices at the same time, increasing network efficiency and throughput.
- Beamforming: Beamforming technology in Wi-Fi 5 helps in focusing the Wi-Fi signal towards the device, rather than broadcasting it in all directions, which enhances the signal strength and reliability.
- Backward Compatibility: Wi-Fi 5 is backward compatible with previous Wi-Fi standards, ensuring that older devices can still connect to Wi-Fi 5 networks.
Wi-Fi 5 represented a substantial step forward in wireless networking technology, offering improved speeds, efficiency, and capacity, particularly for environments with high data demands.
Wi-Fi 6, officially known as IEEE 802.11ax, is the sixth generation of Wi-Fi standards and a significant upgrade over its predecessor, Wi-Fi 5 (802.11ac). Introduced to provide better performance in environments with a lot of connected devices, Wi-Fi 6 offers several improvements:
- Increased Data Rates: Wi-Fi 6 provides higher data rates compared to Wi-Fi 5, thanks to more efficient data encoding and larger channel bandwidth. This results in faster internet speeds and better performance.
- Improved Network Efficiency: One of the key features of Wi-Fi 6 is OFDMA (Orthogonal Frequency Division Multiple Access), which allows one transmission to deliver data to multiple devices at once. This significantly improves efficiency, especially in crowded networks.
- Better Performance in Congested Areas: Wi-Fi 6 shines in areas with many connected devices, such as stadiums, airports, and urban apartments. It reduces latency and improves throughput, making the network more responsive.
- Enhanced Battery Life for Connected Devices: Wi-Fi 6 introduces Target Wake Time (TWT), a feature that schedules communication between the router and devices. This reduces the amount of time devices need to keep their antennas active, conserving battery life.
- Improved Security: Wi-Fi 6 comes with WPA3, the latest Wi-Fi security protocol, which enhances user data protection, especially on public networks.
- Backward Compatibility: Wi-Fi 6 routers and devices are backward compatible with previous Wi-Fi standards, ensuring that older devices can still connect to new networks.
- Wider Channel Bandwidth: It supports 1024-QAM (Quadrature Amplitude Modulation), which increases throughput for emerging, bandwidth-intensive use cases.
- MU-MIMO Enhancements: Multi-user, multiple input, multiple output (MU-MIMO) technology allows more data to be transferred at once and enables an access point to communicate with more than one device simultaneously.
Wi-Fi 6 is designed for the next generation of connectivity, offering faster speeds, greater capacity, and better performance in environments with a lot of wireless devices.
Wi-Fi 7, technically known as 802.11be, is the forthcoming generation of Wi-Fi technology, following Wi-Fi 6 (802.11ax). It is being developed by the IEEE (Institute of Electrical and Electronics Engineers) and is expected to significantly enhance wireless networking performance. Key features and advancements of Wi-Fi 7 include:
- Higher Data Rates: Wi-Fi 7 is anticipated to offer substantially higher maximum data rates than Wi-Fi 6, potentially up to 30-40 Gbps. This is achieved through increased bandwidth and more efficient use of the wireless spectrum.
- Enhanced Bandwidth Utilization: The standard aims to support wider channel bandwidths, up to 320 MHz, and improve the utilization of available frequency bands, including 2.4 GHz, 5 GHz, and 6 GHz.
- Multi-Link Operation (MLO): A significant feature of Wi-Fi 7, MLO allows devices to use multiple bands and channels simultaneously. This can enhance throughput, reduce latency, and improve reliability.
- Advanced Modulation Techniques: Wi-Fi 7 is expected to support 4096-QAM (Quadrature Amplitude Modulation), enabling more bits to be transmitted with each signal, thus increasing the overall data throughput.
- Improved Latency: The new standard aims to significantly reduce latency, which is crucial for applications that require real-time communication, such as online gaming, augmented reality, and virtual reality.
- Increased Network Efficiency: Wi-Fi 7 is designed to be more efficient, especially in environments with many active devices, by utilizing more sophisticated technologies like improved spatial reuse and better scheduling algorithms.
- Backward Compatibility: Like its predecessors, Wi-Fi 7 is expected to be backward compatible with older Wi-Fi standards, ensuring a smooth transition for users upgrading their network hardware.
- Better Power Management: Features like Target Wake Time (TWT) are likely to be enhanced to improve power efficiency for IoT and mobile devices, extending their battery life.
Wi-Fi 7, with its advanced capabilities, is poised to meet the growing demands for higher data rates, lower latency, and more efficient networking in increasingly crowded and diverse wireless environments. As of my last update in April 2023, Wi-Fi 7 is still in the development phase, with its finalization and widespread adoption expected in the following years.
Wi-Fi HaLow, designated as 802.11ah, is a wireless networking protocol developed under the IEEE 802.11 standard. It’s specifically designed for the Internet of Things (IoT) applications. Key features and aspects of Wi-Fi HaLow include:
- Sub-GHz Operation: Unlike traditional Wi-Fi that operates in the 2.4 GHz and 5 GHz bands, Wi-Fi HaLow operates in frequency bands below 1 GHz. This allows for better range and penetration through obstacles like walls and floors.
- Extended Range: Wi-Fi HaLow is known for its long-range capabilities, typically offering coverage over several kilometers. This makes it ideal for IoT applications spread over large areas, like agricultural or industrial environments.
- Low Power Consumption: Devices using Wi-Fi HaLow are designed for low power usage, which is essential for IoT devices, many of which need to operate for years on a small battery.
- High Device Capacity: Wi-Fi HaLow can support thousands of connected devices under a single access point, much more than traditional Wi-Fi. This is particularly important for IoT applications, where many devices are often deployed in a condensed area.
- Use Cases: Wi-Fi HaLow is suited for a range of IoT applications, including smart home and building automation, agricultural and environmental sensors, and industrial monitoring.
- Compatibility and Security: Wi-Fi HaLow retains the core characteristics of the Wi-Fi protocol, including security protocols and ease of integration with existing Wi-Fi technologies.
- Data Rates: While it supports lower data rates compared to conventional Wi-Fi, it’s sufficient for the typical data needs of IoT devices, which usually transmit small amounts of data.
In summary, Wi-Fi HaLow extends the versatility of Wi-Fi to IoT applications, offering solutions for long-range, low-power, and high-density connectivity challenges.
4G (1)
4G LTE Cat-1bis modules are a type of wireless communication module designed for the LTE (Long-Term Evolution) network. They are an enhancement of the original Category 1 (Cat-1) LTE modules and offer some specific features and improvements. Here are the key aspects of 4G LTE Cat-1bis modules:
- Enhanced Data Rates: While standard Cat-1 modules support data rates up to 10 Mbps for download and 5 Mbps for upload, Cat-1bis modules are designed to provide improved data rates. The exact speeds can vary, but they are generally higher than the basic Cat-1 specifications.
- Power Efficiency: Cat-1bis modules are designed to be more power-efficient compared to their predecessors. This makes them suitable for IoT devices that require a balance between moderate data rate requirements and long battery life.
- Lower Complexity: These modules are less complex than higher category LTE modules (such as Cat-4 or Cat-6), which makes them a cost-effective solution for applications that do not require very high data rates.
- Applications: 4G LTE Cat-1bis modules are ideal for a range of IoT and M2M (Machine to Machine) applications that require better connectivity than 2G or 3G but do not necessarily need the high speeds offered by more advanced LTE categories. These include telematics, smart metering, security systems, remote monitoring, and other IoT applications.
- Backward Compatibility: Like other LTE technologies, Cat-1bis modules are typically backward compatible with existing 2G and 3G networks, ensuring connectivity even in areas where 4G coverage is not available.
- VoLTE Support: Some Cat-1bis modules support Voice over LTE (VoLTE), which can be a critical feature for certain applications that require voice communication capabilities.
In summary, 4G LTE Cat-1bis modules provide a balanced solution for IoT and M2M applications, offering enhanced data rates and power efficiency compared to standard Cat-1 LTE modules, without the complexity and cost of higher category LTE technologies.
5G (9)
5G Advanced, often referred to as “5G-Advanced” or “5.5G,” represents the evolution and enhancement of 5G technology. It is considered the next phase in 5G development, following the initial release of 5G standards (Release 15 and Release 16 by the 3GPP). 5G Advanced aims to expand and improve upon the capabilities of 5G to meet growing demands and emerging technological trends. Key aspects of 5G Advanced include:
- Enhanced Performance: 5G Advanced aims to further increase data rates, reduce latency, and improve network efficiency beyond the initial specifications of 5G.
- Improved Network Capacity and Coverage: It focuses on enhancing network capacity to support an even larger number of connected devices, as well as improving coverage, particularly in challenging environments.
- Advanced Network Features: This includes more advanced forms of network slicing, improved Massive MIMO (Multiple Input Multiple Output) technologies, and enhancements in beamforming for better signal direction and strength.
- Integration with Emerging Technologies: 5G Advanced is expected to better integrate with technologies like Artificial Intelligence (AI), Machine Learning (ML), and edge computing, offering more intelligent and responsive network solutions.
- Support for Diverse Applications: While 5G already supports a wide range of applications, 5G Advanced will further expand capabilities in areas such as the Internet of Things (IoT), ultra-reliable low-latency communications (URLLC), and enhanced mobile broadband (eMBB).
- Sustainability and Energy Efficiency: A focus on sustainability, with improvements in energy efficiency, is a key aspect of 5G Advanced, addressing the environmental impact of expanding network infrastructures.
- Research and Standardization: 5G Advanced is currently in the research and standardization phase, with industry and academia collaborating to define its features and capabilities.
5G Advanced represents the continuous evolution of 5G networks, aiming to accommodate the ever-increasing demand for data and connectivity and to enable new applications and technologies that require more advanced network capabilities.
5G Convergence refers to the integration and unification of various technologies, services, and network architectures under the umbrella of 5G wireless technology. This convergence aims to create a more seamless and efficient telecommunications ecosystem. Key aspects of 5G Convergence include:
- Unified Network Architecture: 5G Convergence involves integrating different types of networks, such as cellular, Wi-Fi, and satellite, into a unified system. This allows for more efficient resource management and service delivery.
- Integration of Services: Convergence in 5G isn’t just about network technologies; it also includes the integration of various services like voice, data, and multimedia, providing a comprehensive and seamless user experience.
- IoT and Industrial Integration: 5G Convergence is crucial in the integration of IoT devices and industrial applications, enabling seamless communication between a vast array of devices and systems.
- Network Slicing: A key feature of 5G, network slicing allows the creation of multiple virtual networks over a single physical network infrastructure. This enables the tailored provisioning of network resources for different applications and services.
- Enhanced Data Processing: With the convergence of edge computing and 5G, data processing becomes more efficient. Data can be processed closer to where it is generated, reducing latency and improving response times.
- Support for Diverse Applications: 5G Convergence supports a wide range of applications, from high-speed mobile broadband to mission-critical communication and massive IoT deployments.
- Standardization and Interoperability: Ensuring interoperability and compliance with global standards is essential in 5G Convergence, to enable seamless communication across devices and networks.
- Advanced Technologies Synergy: 5G Convergence brings together advancements like AI, big data analytics, and cloud computing, leveraging these technologies to enhance network performance and user experience.
5G Convergence represents a transformation in how communication networks are built and operated, offering a more integrated, flexible, and efficient approach to meet the diverse demands of modern digital society.
5G Fixed Wireless Access (FWA) is a method of providing wireless broadband internet services to homes and businesses using 5G cellular network technology. It is an alternative to traditional wired broadband like DSL, cable, or fiber optics. Here are key aspects of 5G FWA:
- Use of 5G Technology: 5G FWA utilizes the high-speed and low-latency capabilities of the 5G network to deliver internet services.
- Wireless Connectivity: Unlike traditional broadband that requires physical wiring, FWA uses wireless signals to connect users to the internet. This can significantly reduce the need for extensive physical infrastructure.
- Deployment Ease: FWA is particularly beneficial in areas where laying cables is challenging or not cost-effective. It allows for rapid deployment of broadband services in rural or underserved areas.
- High-Speed Internet: With 5G technology, FWA can offer comparable, and in some cases, superior speeds to wired broadband solutions, suitable for high-bandwidth applications like streaming, gaming, and video conferencing.
- Network Infrastructure: The setup typically involves a 5G modem or router at the user’s location, which communicates with the nearest 5G cell tower to provide internet connectivity.
- Cost-Effectiveness: For network providers, FWA can be a more cost-effective way to expand broadband access, especially in less densely populated areas.
- Improved Capacity and Range: Leveraging advanced 5G technologies like beamforming and Massive MIMO, FWA can offer improved capacity and range compared to earlier wireless technologies.
5G FWA is seen as a key component in the broader rollout of 5G, offering a flexible and efficient way to expand broadband access and bridge the digital divide, particularly in regions where wired infrastructure is lacking or insufficient.
5G Massive IoT refers to the application of 5G technology to massively connect a large number of Internet of Things (IoT) devices. This concept is part of the broader vision of 5G networks, which aim to provide not just faster internet speeds for smartphones but also to enable the interconnectivity of billions of devices. Here are key aspects of 5G Massive IoT:
- High Device Connectivity: One of the primary goals of 5G Massive IoT is to support an extremely large number of connected devices per square kilometer, far exceeding the capacity of previous cellular technologies.
- Low Power Consumption: 5G Massive IoT focuses on providing connectivity to devices that require low power consumption, enabling devices to operate for years on a single battery charge. This is crucial for sensors and devices in remote or hard-to-reach locations.
- Wide Range and Deep Coverage: 5G technology aims to offer enhanced coverage that can reach challenging areas, such as deep indoors or in rural locations, making it suitable for a wide range of IoT applications.
- Small Data Packets: Massive IoT devices typically transmit small amounts of data infrequently. 5G networks are designed to efficiently handle such small data packets, optimizing network usage and performance.
- Diverse Applications: Applications of 5G Massive IoT are diverse and include smart cities, industrial IoT, environmental monitoring, agriculture, smart buildings, and more.
- Integration with Other Technologies: 5G Massive IoT is expected to work in tandem with other technologies like edge computing and AI to process and manage the vast amounts of data generated by IoT devices.
- Enhanced IoT Capabilities: Beyond connectivity, 5G Massive IoT aims to enhance capabilities such as device-to-device communication, real-time data analytics, and automated decision-making processes.
In summary, 5G Massive IoT represents a significant leap in the capability to connect a vast number of IoT devices, enabling new applications and efficiencies across various industries, and is a critical component of the evolving 5G landscape.
5G RedCap (Reduced Capability) is a new feature introduced in the 3rd Generation Partnership Project (3GPP) Release 17. It is designed to cater to devices that require higher capabilities than those offered by LTE-M or NB-IoT (both are narrowband IoT technologies), but do not need the full capabilities of standard 5G devices. This makes 5G RedCap particularly suitable for a specific segment of IoT and industrial applications. Key aspects of 5G RedCap include:
- Lower Device Complexity: 5G RedCap aims to reduce the complexity and cost of devices compared to full-featured 5G devices. This is achieved by scaling down certain aspects of the 5G technology.
- Moderate Data Rates: While offering lower data rates than the full 5G standard, 5G RedCap still provides higher data rates than narrowband IoT technologies, making it suitable for applications that require moderate bandwidth.
- Energy Efficiency: With its reduced complexity, 5G RedCap also aims to improve energy efficiency, which is crucial for battery-powered IoT devices.
- Broad Applications: This technology is ideal for a range of IoT applications, including wearables, industrial sensors, and certain types of smart meters that need more capability than NB-IoT or LTE-M but do not require the high data rates and full capabilities of 5G.
- Network Compatibility: 5G RedCap is designed to be compatible with existing 5G networks, enabling seamless integration with the current infrastructure.
- Balanced Performance: The key advantage of 5G RedCap is its balanced performance, offering better capabilities than narrowband technologies while avoiding the complexity and cost of full 5G.
In summary, 5G RedCap represents an important step in the evolution of 5G and IoT, bridging the gap between narrowband IoT technologies and full 5G, and providing a more cost-effective and efficient solution for a wide range of IoT applications.
CBRS (Citizens Broadband Radio Service) in the context of 5G-Advanced refers to an innovative approach in wireless communication where the CBRS spectrum is utilized for advanced 5G applications. CBRS operates in the 3.5 GHz band (3550 MHz to 3700 MHz) in the United States and is designed to offer a shared spectrum model. This approach is significant in the evolution of 5G networks for several reasons:
- Shared Spectrum Access: CBRS uses a three-tiered shared spectrum access system, allowing for efficient use of the 3.5 GHz band. This system includes Incumbent Access, Priority Access, and General Authorized Access.
- Enhanced Capacity and Coverage: By leveraging the CBRS band, 5G-Advanced networks can enhance capacity and coverage, particularly in densely populated areas or for enterprise use cases.
- Flexibility and Cost-Effectiveness: CBRS offers a more flexible and cost-effective way for organizations to deploy private 5G networks, as it reduces the need for purchasing exclusive spectrum licenses.
- Innovation in Wireless Services: The utilization of CBRS in 5G-Advanced paves the way for innovative wireless services and applications, including IoT deployments, industrial automation, and enhanced mobile broadband.
- Improved Network Performance: The CBRS band is well-suited for 5G use due to its balance between coverage and capacity, making it ideal for a variety of applications from urban to rural deployments.
- Regulatory Framework: The Federal Communications Commission (FCC) has established rules for CBRS, promoting efficient use of the spectrum while protecting incumbent users.
- Compatibility with Existing Technology: CBRS can be integrated with existing LTE and 5G NR technology, allowing for seamless adoption and integration into current network infrastructures.
CBRS 5G-Advanced represents a significant step in diversifying the spectrum usage for 5G, offering new opportunities for network operators, enterprises, and other entities to deploy flexible and efficient 5G solutions.
Enhanced Mobile Broadband (eMBB) is one of the three primary use case categories defined for 5G networks by the 3rd Generation Partnership Project (3GPP), alongside Ultra-Reliable Low-Latency Communications (URLLC) and Massive Machine Type Communications (mMTC). eMBB focuses on providing significantly higher data rates and greater capacity compared to previous mobile network generations. Key aspects of eMBB include:
- High Data Speeds: eMBB aims to deliver peak data rates up to several gigabits per second (Gbps), which is a substantial increase over 4G data rates. This enables applications that require high bandwidth, such as high-definition video streaming, augmented reality, and virtual reality.
- Improved Network Capacity: eMBB is designed to support a higher number of connected devices and higher throughput per area, which is essential for crowded urban areas and for events with high user density.
- Enhanced User Experience: The increased speed and capacity contribute to a significantly enhanced user experience, with faster download and upload speeds, higher quality video content, and more reliable connectivity.
- Broadband Everywhere: eMBB also aims to provide high-speed mobile broadband services in areas where fixed broadband is unavailable or limited, effectively bridging the digital divide.
- Support for Diverse Applications: While eMBB is primarily associated with consumer applications like streaming and gaming, it also supports a wide range of business applications, including cloud services and teleconferencing.
- Advanced Antenna Technologies: The deployment of eMBB involves advanced technologies such as Massive MIMO (Multiple Input Multiple Output) and beamforming, which are key to achieving the high data rates and capacity.
- Spectrum Utilization: eMBB makes use of a wide range of frequency bands, from sub-6 GHz for wide coverage to millimeter-wave bands for high-capacity, short-range coverage.
eMBB represents a significant evolution in wireless broadband capabilities, setting the foundation for a new generation of mobile applications and services enabled by 5G technology.
Massive Machine Type Communications (mMTC) is one of the three main use case categories defined for 5G networks by the 3rd Generation Partnership Project (3GPP), alongside Enhanced Mobile Broadband (eMBB) and Ultra-Reliable Low-Latency Communications (URLLC). mMTC is focused on enabling large-scale communication between devices, typically for IoT applications. Key aspects of mMTC include:
- Large-Scale Connectivity: mMTC is designed to support a vast number of connected devices, potentially in the order of millions per square kilometer. This is crucial for IoT applications that require extensive sensor networks.
- Low Power Requirement: Devices used in mMTC networks are typically designed to be low-power, allowing them to operate for years on a small battery, which is essential for IoT devices deployed in remote or hard-to-reach areas.
- Small Data Packets: mMTC is optimized for the transmission of small, infrequent data packets, which is characteristic of many IoT and sensor applications.
- High Density and Scalability: mMTC networks are designed to handle high device densities, ensuring reliable communication even in environments with a large number of IoT devices.
- Cost-Effective Solutions: The focus is on providing cost-effective connectivity solutions, enabling the deployment of IoT devices and sensors on a large scale without significantly increasing costs.
- Applications: mMTC is applicable in various sectors including smart cities, industrial monitoring, agriculture, environmental sensing, and smart homes, where a large number of devices need to be connected.
- Network Efficiency: Strategies like network slicing are used to efficiently manage and prioritize network resources for mMTC traffic.
mMTC is a key component of the 5G landscape, enabling the widespread and efficient connectivity of IoT devices and facilitating the growth of smart environments and applications.
URLLC, or Ultra-Reliable Low-Latency Communications, is a service category in 5G networks designed to support applications that require very high reliability and extremely low latency. It is one of the three primary use case categories defined for 5G, alongside Enhanced Mobile Broadband (eMBB) and Massive Machine Type Communications (mMTC). Key aspects of URLLC include:
- Low Latency: URLLC aims to achieve end-to-end latency in the order of milliseconds, significantly lower than what is possible in previous generation networks. This is crucial for applications requiring real-time responses.
- High Reliability: URLLC provides highly reliable communication links, with success rates as high as 99.999% for data transmission. This level of reliability is essential for critical applications where errors or delays could have severe consequences.
- Critical Applications Support: URLLC is tailored for use cases such as autonomous vehicles, industrial automation, remote surgery, and other applications where instantaneous, reliable communication is vital.
- Network Slicing: Leveraging network slicing in 5G, specific slices of the network can be allocated for URLLC services, ensuring dedicated resources and prioritization over other types of network traffic.
- Advanced Technologies: The implementation of URLLC involves various advanced technologies, including edge computing, advanced antenna technologies like beamforming, and enhanced modulation techniques to minimize transmission delays and errors.
- Spectrum Efficiency: URLLC requires efficient use of the spectrum to meet its stringent latency and reliability requirements, often using techniques like OFDMA (Orthogonal Frequency Division Multiple Access).
- Standardization: URLLC is part of the 3GPP standards for 5G (starting from Release 15 onwards), which define the technical aspects and requirements for deploying URLLC services.
URLLC is a cornerstone for enabling a wide range of future technologies and applications that depend on rapid, reliable wireless communication, and is a key differentiator of 5G networks from their predecessors.
IoT (4)
The SGP.32 eSIM IoT Technical Specification refers to a set of standards and guidelines developed by the GSMA (Global System for Mobile Communications Association) for the implementation of eSIM technology in IoT (Internet of Things) devices. “eSIM” stands for “Embedded Subscriber Identity Module,” and it represents a significant advancement in SIM card technology. The SGP.32 specification outlines how eSIMs should be integrated and managed within IoT applications. Key aspects of the SGP.32 eSIM IoT Technical Specification include:
- eSIM Profile Management: The specification details how eSIM profiles can be remotely managed and provisioned. This includes downloading, enabling, disabling, and deleting profiles on the eSIM.
- Interoperability: Ensuring that eSIMs and related management systems are interoperable across different manufacturers and network operators is a core focus of the specification.
- Security: SGP.32 includes robust security guidelines for the protection of data on eSIMs. This encompasses secure transmission of eSIM profiles and safeguarding sensitive information.
- Remote Provisioning Architecture for Embedded UICC: The specification provides a detailed framework for the remote provisioning and management of eSIMs in IoT devices, ensuring consistency and reliability in the deployment of eSIM technology.
- Lifecycle Management: It addresses the entire lifecycle of an eSIM, from initial deployment to end-of-life, including updates and maintenance procedures.
- Scalability and Flexibility: The standards are designed to be scalable and flexible to accommodate a wide range of IoT devices and applications, from small-scale consumer products to large industrial systems.
- Integration with IoT Platforms: The specification also considers how eSIM technology integrates with broader IoT platforms and ecosystems, including cloud services and analytics tools.
The SGP.32 eSIM IoT Technical Specification is instrumental in advancing the use of eSIM technology in the IoT space, offering a more flexible, secure, and efficient approach to device connectivity and management.
Narrowband IoT (NB-IoT) is a Low Power Wide Area Network (LPWAN) radio technology standard developed to enable a wide range of devices and services to be connected using cellular telecommunication bands. NB-IoT is one of several standards developed to meet the growing needs of IoT (Internet of Things) applications. Here are some key aspects of NB-IoT:
- Low Power Usage: NB-IoT devices are designed for low power consumption, allowing them to operate for years on a single battery charge. This is ideal for IoT devices that need to be deployed for long periods without maintenance.
- Extended Coverage: NB-IoT provides improved indoor and rural coverage compared to traditional mobile networks. It achieves this by using a simpler waveform that can penetrate deep into buildings and underground areas.
- Narrow Bandwidth: As the name suggests, NB-IoT operates on a narrow bandwidth of just 200 kHz. This narrowband technology is beneficial for applications that require small amounts of data to be transmitted infrequently.
- Cost-Effective: The infrastructure required for NB-IoT is less expensive compared to broader bandwidth cellular networks. This makes it a cost-effective solution for deploying large-scale IoT networks.
- High Connection Density: NB-IoT supports a high number of connected devices per cell. This makes it suitable for applications where many devices need to be interconnected in a condensed area.
- Applications: Typical applications of NB-IoT include smart meters, smart parking, asset tracking, environmental monitoring, and smart agriculture.
- Standardization and Compatibility: NB-IoT is a standardized technology (by 3GPP) and is backed by major telecommunications operators. It is compatible with existing cellular network infrastructure, allowing for easy integration and deployment.
In summary, Narrowband IoT offers a highly efficient, cost-effective, and standardized way to connect a large number of devices over wide areas, making it an integral part of the IoT ecosystem.
The Cellular IoT Ecosystem refers to the comprehensive environment that encompasses technologies, devices, networks, and services enabling cellular connectivity for the Internet of Things (IoT). This ecosystem is built around the use of cellular networks (like LTE, 5G) to connect IoT devices. Here are key components and aspects of the Cellular IoT Ecosystem:
- Cellular Networks: The foundation of this ecosystem is cellular networks, including LTE (4G), 5G, and specialized subsets like NB-IoT and LTE-M, which are designed for low-power, wide-area IoT applications.
- IoT Devices and Sensors: These are the endpoints in the ecosystem, ranging from simple sensors to complex machines. They collect and transmit data over cellular networks.
- Connectivity Management: Tools and platforms that manage and control the connectivity of IoT devices, ensuring seamless communication, security, and data flow.
- Data Processing and Analytics: Once data is transmitted over the network, it is processed and analyzed. This can occur in cloud-based platforms or edge computing devices.
- Applications and Services: The ecosystem is driven by a vast range of applications across various industries such as healthcare, agriculture, smart cities, industrial automation, and more.
- Security: As these devices often collect and transmit sensitive data, security is a crucial component, including encryption, network security protocols, and secure device management.
- Regulatory Framework: Compliance with regional and international regulations and standards is essential for operation within legal and ethical guidelines.
- Service Providers and Ecosystem Partners: The ecosystem involves collaboration between hardware manufacturers, software developers, network operators, service providers, and other stakeholders.
- Innovation and Development: Continuous innovation is key, with ongoing development in areas like 5G technology, low-power wide-area network solutions, and enhanced security protocols.
In summary, the Cellular IoT Ecosystem represents the integration of multiple technologies and components, working together to enable a wide range of IoT applications through cellular connectivity. This ecosystem is evolving rapidly, driven by advancements in cellular technology and the increasing demand for IoT solutions.
Wi-Fi Sensing technology, also known as Wi-Fi positioning or Wi-Fi based sensing, is an innovative use of Wi-Fi signals to detect and interpret movements or changes in the environment. This technology does not rely on traditional video or infrared sensors but uses the characteristics of Wi-Fi signals such as signal strength, phase, and timing. Here are some key aspects of Wi-Fi Sensing technology:
- Movement Detection: Wi-Fi Sensing can detect movement in an environment by analyzing disruptions or changes in Wi-Fi signal patterns caused by motion.
- Location Tracking: It can be used to track the location of devices or people within a Wi-Fi network’s range, based on how their presence affects Wi-Fi signals.
- Privacy-Friendly: Since it doesn’t rely on cameras, Wi-Fi Sensing is considered more privacy-friendly for monitoring and security applications, as it doesn’t capture visual images.
- Smart Home Applications: In smart homes, Wi-Fi Sensing can be used for applications like security alarms, monitoring the well-being of residents, automating lighting or heating based on occupancy, and detecting unusual activities.
- Health Monitoring: It has potential applications in health monitoring, such as fall detection for the elderly or monitoring breathing patterns during sleep.
- Retail and Business Analytics: Businesses can use Wi-Fi Sensing for customer movement and behavior analytics, helping to understand customer preferences and enhance the in-store experience.
- Integration with Existing Hardware: One of the advantages of Wi-Fi Sensing is that it can often be integrated into existing Wi-Fi infrastructure with software updates, reducing the need for additional hardware.
- Emerging Technology: Wi-Fi Sensing is an emerging technology and is continually being developed to improve accuracy, reliability, and the range of applications.
Wi-Fi Sensing technology leverages the widespread availability of Wi-Fi and provides a novel way to gather environmental data without additional hardware, opening up new possibilities in smart environments, security, healthcare, and retail analytics.
LTE (2)
LTE (Long-Term Evolution) networks represent a standard for wireless broadband communication. They are designed to increase the capacity and speed of wireless data networks. LTE is often referred to as 4G LTE and is a major step up from 3G networks in terms of speed and efficiency. Here are key aspects of LTE networks:
- High-Speed Data Transmission: LTE networks provide significantly higher data speeds for both downloading and uploading compared to earlier mobile networks like 3G. This enables faster internet browsing, streaming of high-definition videos, and quicker download times.
- Improved Capacity and Efficiency: LTE networks are more efficient at handling data, voice, and video traffic, leading to more reliable service, even during peak times or in crowded areas.
- Lower Latency: LTE offers reduced latency, which is the time taken for a data packet to travel from its source to its destination. This results in improved performance for applications that require real-time data transmission, like online gaming and video conferencing.
- Enhanced Bandwidth: LTE networks use a wider radio spectrum bandwidth, providing more space for data traffic and thereby improving network capacity and speed.
- Better Coverage: While the extent of coverage depends on the network provider, LTE networks generally provide better and more extensive coverage compared to their 3G counterparts.
- Evolution to LTE-Advanced: LTE-Advanced is an upgrade to the standard LTE technology, offering even higher speeds and capacity. It includes features like carrier aggregation (combining multiple LTE carriers), higher-order MIMO (Multiple Input Multiple Output), and enhanced use of spectrum.
- Global Adoption: LTE is widely adopted around the world, enabling global roaming for LTE-equipped devices, subject to the compatibility of frequency bands between different regions.
LTE networks have been instrumental in driving the growth of mobile internet and are the backbone of modern mobile communication, paving the way for the next generation of wireless technology, including 5G networks.
LTE-Advanced, also known as 4G+, is an enhancement to the original LTE (Long-Term Evolution) technology. It was standardized by the 3rd Generation Partnership Project (3GPP) as part of its Release 10 and beyond. LTE-Advanced aims to provide faster and more efficient data rates, enhanced performance, and better user experience compared to its predecessor, LTE. Key features and improvements of LTE-Advanced include:
- Carrier Aggregation (CA): One of the most significant enhancements in LTE-Advanced. Carrier Aggregation allows the network to combine multiple LTE carriers, boosting data rates by increasing the bandwidth available for data transmission.
- Higher Order MIMO (Multiple Input Multiple Output): LTE-Advanced supports more antennas than LTE, allowing for higher order MIMO configurations. This increases the potential data rate and capacity of the network, especially in densely populated areas.
- Enhanced Use of Spectrum: LTE-Advanced can operate over a wider range of frequency bands and bandwidths, from 1.4 MHz up to 100 MHz. This flexibility enables better use of available spectrum and improves network performance.
- Improved Network Efficiency: Enhanced inter-cell interference coordination (eICIC) and Coordinated Multi-Point (CoMP) operations are introduced to improve network efficiency, especially at cell edges and in densely populated urban areas.
- Advanced Modulation Techniques: LTE-Advanced employs advanced modulation techniques, like 256-QAM (Quadrature Amplitude Modulation), enabling higher throughput under suitable conditions.
- Backward Compatibility: LTE-Advanced is backward compatible with LTE, meaning devices and networks can switch between LTE and LTE-Advanced depending on availability and network conditions.
- Application Scenarios: LTE-Advanced is suitable for high-demand applications such as high-definition video streaming, large-scale online gaming, and high-speed mobile internet access.
In summary, LTE-Advanced represents a significant step forward in mobile network technology, offering increased speed, improved efficiency, and better overall performance, setting the stage for the transition to even more advanced technologies like 5G.
Networks (5)
Brownfield networks refer to existing telecommunications networks that have been previously developed and deployed, often using legacy technologies and equipment. These networks contrast with “greenfield” networks, built from scratch using the latest technologies and standards. Key aspects of brownfield networks include:
- Legacy Systems: Brownfield networks often include older technologies that may not be compatible with the latest standards or innovations. This includes older generations of wireless technology, like 2G and 3G, or traditional wired networks.
- Integration Challenges: Integrating new technologies or upgrades into brownfield networks can be challenging due to compatibility issues with legacy systems and equipment.
- Cost Considerations: While upgrading brownfield networks can be cost-effective compared to building new infrastructure, the process of modernization can be complex and resource-intensive.
- Maintenance and Operations: Maintaining brownfield networks involves managing aging infrastructure, which may require more frequent repairs and upkeep.
- Migration to Newer Technologies: Transitioning from brownfield to more modern network infrastructures, like 4G, 5G, or fiber-optic networks, often requires strategic planning and phased implementation to minimize service disruptions.
- Customer Base: Brownfield networks typically have an existing customer base, which can be an advantage in terms of market presence, but also poses the challenge of ensuring service continuity during upgrades.
- Regulatory Compliance: Ensuring that brownfield networks comply with current regulatory standards is crucial, especially when integrating new technologies or services.
Understanding and effectively managing brownfield networks is essential for telecommunications operators, especially in the context of ongoing industry advancements and the need for digital transformation.
Greenfield networks refer to telecommunications networks built from scratch with no constraints from existing systems or infrastructures. This term is often used in contrast to “brownfield” networks, which involve upgrading or integrating with existing network infrastructure. Key aspects of greenfield networks include:
- Latest Technologies: Greenfield projects offer the opportunity to deploy the latest technologies, such as 5G in wireless networks or advanced fiber optics in wired networks, without the limitations of legacy systems.
- Design and Implementation Flexibility: Building a greenfield network allows for more flexibility in design and implementation, enabling a more optimized and efficient network architecture that is future-proof.
- Cost Considerations: While the initial investment for a greenfield project might be high due to the need for new infrastructure, it can be more cost-effective in the long term due to lower maintenance and operational costs.
- Faster Deployment of Advanced Services: Greenfield networks can more rapidly deploy advanced services and applications, benefiting from the inherent efficiencies and capabilities of the latest technologies.
- Challenges in Market Penetration: For new operators, establishing a greenfield network can be challenging in terms of gaining market share and attracting customers, especially in regions with established competitors.
- Regulatory Compliance: Greenfield projects must comply with all current regulatory standards and requirements, which can vary depending on the region and the type of services offered.
- Sustainability and Environmental Considerations: New network deployments can incorporate sustainability practices and eco-friendly technologies from the outset.
Greenfield networks represent an ideal scenario for deploying the most advanced telecommunications technologies and can set the foundation for innovative services and applications. They are particularly relevant in areas without existing telecommunications infrastructure or where the existing infrastructure is insufficient to meet current and future demands.
The official release dates of Wi-Fi 4, Wi-Fi 5, Wi-Fi 6, and Wi-Fi 7 (as per the IEEE standardization and Wi-Fi Alliance naming conventions) are as follows:
- Wi-Fi 4 (IEEE 802.11n):
- Standard Finalized: October 2009
- Wi-Fi 4 is the designation given to the IEEE 802.11n standard, which significantly improved upon previous Wi-Fi standards by introducing technologies like MIMO and increased data rates.
- Wi-Fi 5 (IEEE 802.11ac):
- Standard Finalized: January 2014 (Wave 1), December 2013 (Wave 2)
- Wi-Fi 5 refers to the IEEE 802.11ac standard, which enhanced Wi-Fi performance further by introducing features like wider channel bandwidth and support for additional spatial streams.
- Wi-Fi 6 (IEEE 802.11ax):
- Standard Finalized: September 2019
- Wi-Fi 6, known technically as IEEE 802.11ax, brought significant advancements in efficiency, especially in crowded environments, and introduced technologies like OFDMA and Target Wake Time (TWT).
- Wi-Fi 7 (IEEE 802.11be):
- Expected Finalization: As of my last update in April 2023, Wi-Fi 7 (IEEE 802.11be) was still in development, with finalization expected around 2024.
- Wi-Fi 7 is expected to offer further improvements in terms of data rates, latency, and efficiency, continuing the evolution of Wi-Fi technology.
These release dates mark important milestones in the development of Wi-Fi technology, with each new generation bringing enhancements that have enabled faster speeds, greater capacity, and more efficient network performance.
Roaming service refers to the ability of a cell phone or mobile device user to automatically make and receive voice calls, send and receive data, or access other services when traveling outside the geographical coverage area of their home network, by using a visited network. This service is essential for maintaining connectivity when users are in areas not served by their carrier’s regular network. Key aspects of roaming services include:
- Types of Roaming:
- Domestic Roaming: Occurs when a user connects to another operator’s network within their home country.
- International Roaming: Involves using a mobile device on a foreign operator’s network while traveling abroad.
- Roaming Agreements: Mobile operators form agreements with other operators to provide roaming services to their subscribers. These agreements cover aspects like service standards, pricing, and data exchange.
- Seamless Connectivity: Roaming is designed to provide seamless service, with users able to use their mobile phones for calls, text messages, and data services just as they do at home.
- Charges and Tariffs: Roaming often incurs additional charges, which can vary significantly depending on the operators involved and the user’s service plan. International roaming, in particular, can be expensive.
- SIM Card and Network Compatibility: Effective roaming depends on the compatibility of the user’s mobile device and SIM card with the visited network, particularly in terms of supported frequency bands and network technology.
- Roaming Partners and Coverage: Mobile operators typically publish lists of their roaming partners and the countries where roaming services are available.
- Regulatory Aspects: In some regions, like the European Union, regulations have been put in place to control roaming charges and protect consumers from high fees.
- Data Roaming: This allows users to access the internet and use data-driven services. Data roaming can be particularly costly, and users often have the option to disable it.
Roaming services are a critical aspect of global telecommunications, enabling users to stay connected while traveling outside their home network’s coverage area.
GSM (Global System for Mobile Communications) is a standard developed by the European Telecommunications Standards Institute (ETSI) to describe the protocols for second-generation (2G) digital cellular networks used by mobile devices such as phones and tablets. Introduced in the 1990s, GSM was a major leap in mobile communication technology. Key aspects of GSM include:
- Digital Communication: GSM marked the transition from analog first-generation (1G) networks to digital, significantly improving voice quality, security, and capacity.
- Global Standard: As its name suggests, GSM became a global standard for mobile communication, facilitating international roaming and compatibility.
- Network Components: GSM networks consist of key subsystems like the Base Station Subsystem (BSS), Network and Switching Subsystem (NSS), and the Operations and Support Subsystem (OSS).
- SIM Cards: GSM introduced the use of SIM (Subscriber Identity Module) cards, which store subscriber data and facilitate mobile device identification and authentication on the network.
- Data Services: Besides voice communication, GSM supports data services such as SMS (Short Message Service) and later, GPRS (General Packet Radio Services) for basic internet connectivity.
- Encryption and Security: GSM networks employ encryption to secure voice and data communication, enhancing privacy and security.
- Frequency Bands: GSM operates in multiple frequency bands, like 900 MHz and 1800 MHz in Europe and 850 MHz and 1900 MHz in the Americas, catering to different regional requirements.
GSM set the foundation for modern mobile communication and led to the development of more advanced technologies like 3G (UMTS) and 4G (LTE).
Organizations (5)
Mobile operators, also known as mobile network operators (MNOs), are companies that provide wireless voice and data communication services to mobile device users. They are an essential part of the telecommunications industry. Key aspects of mobile operators include:
- Network Infrastructure: Mobile operators own or control access to the network infrastructure necessary to provide services to mobile phone subscribers. This includes cell towers, networking equipment, and back-end systems.
- Service Provisioning: They offer various services such as voice calls, text messaging (SMS), multimedia messaging (MMS), and internet access. With advancements in technology, services have expanded to include mobile broadband, streaming, and more.
- Spectrum Licensing: Mobile operators typically acquire licenses to operate in specific frequency bands from government regulatory bodies. This spectrum is crucial for transmitting and receiving wireless signals.
- Technology Adoption: They are responsible for upgrading their networks to support newer technologies (e.g., transitioning from 3G to 4G LTE, and now to 5G), enhancing speed, capacity, and service quality.
- Subscriber Management: Mobile operators manage customer relationships, including billing, customer service, and offering various plans and packages to cater to different user needs.
- Regulatory Compliance: They must comply with the regulations and policies set by telecommunications regulatory authorities, which may include aspects like service quality, fair competition, and emergency services.
- Roaming Services: Mobile operators often establish agreements with operators in other regions or countries to provide service to their subscribers when they are outside their home network (roaming).
- Value-Added Services: Besides basic communication services, many operators offer additional services like music streaming, video content, cloud storage, and digital payments.
Mobile operators play a crucial role in connecting people and devices, driving innovation in the telecommunications sector, and facilitating the growth and adoption of new mobile technologies.
The European Telecommunications Standards Institute (ETSI) is an independent, non-profit standardization organization for the telecommunications industry in Europe, with a worldwide influence. ETSI plays a significant role in developing global standards for Information and Communication Technologies (ICT), including fixed, mobile, radio, converged, broadcast, and internet technologies. Key aspects of ETSI include:
- Standard Development: ETSI is responsible for creating internationally-applicable standards across a wide range of telecommunications and ICT services and technologies.
- Global Influence: While ETSI is focused on Europe, its standards are often adopted worldwide. The GSM standard developed by ETSI is a prime example of its global impact.
- Membership: ETSI’s members include manufacturers, network operators, service providers, research bodies, and national administrations from across the globe.
- Collaboration with Other Bodies: ETSI collaborates with other standardization organizations like ITU (International Telecommunication Union) and 3GPP (3rd Generation Partnership Project) to ensure global alignment and interoperability of standards.
- Innovation and Technology Development: ETSI is involved in emerging and future-oriented technologies, playing a key role in areas like 5G, IoT (Internet of Things), and cybersecurity.
- Standards for New Technologies: ETSI has been instrumental in developing standards for various new technologies, including those related to network functions virtualization (NFV), software-defined networking (SDN), and more.
ETSI’s work ensures compatibility and interoperability of systems, which is vital for the global telecommunications industry, fostering innovation and facilitating seamless communication and connectivity.
The GSMA (Global System for Mobile Communications Association) is an industry organization that represents the interests of mobile network operators worldwide. Established in 1987, it plays a central role in shaping the future of mobile communications and the wider mobile ecosystem. Here are some key aspects of the GSMA:
- Membership and Representation: The GSMA has a large and diverse membership that includes nearly 800 mobile operators and more than 300 companies in the broader mobile ecosystem, including handset manufacturers, software companies, equipment providers, and internet companies.
- Standards and Policies: One of its main roles is to develop and promote mobile industry standards and policies. The GSMA works closely with standardization bodies, governments, and other organizations to foster a collaborative environment for standard development.
- Mobile World Congress: The GSMA is perhaps best known for organizing the Mobile World Congress (MWC) events, which are among the largest annual exhibitions and conferences in the mobile industry, held in different locations around the world.
- Advocacy and Research: The organization advocates on behalf of its members on a range of issues, from regulatory and public policy to technology and health matters. It also conducts research and publishes reports on various aspects of the mobile industry.
- Sustainability and Social Impact: The GSMA is involved in initiatives that use mobile technology for positive social and economic impact. This includes efforts in areas such as environmental sustainability, digital inclusion, and emergency response.
- 5G Development: The GSMA plays a significant role in the development and adoption of 5G technology, collaborating with industry stakeholders to establish standards and ensure a smooth rollout of 5G networks.
In summary, the GSMA is a key global organization in the mobile communications industry, facilitating collaboration, innovation, and strategic development to benefit mobile operators and the wider mobile ecosystem.
The Federal Communications Commission (FCC) is an independent agency of the United States government created by statute (47 U.S.C. § 151 and 47 U.S.C. § 154) to regulate interstate communications by radio, television, wire, satellite, and cable. It plays a key role in managing communication technologies and services in the U.S. Key aspects of the FCC include:
- Regulation and Oversight: The FCC regulates all non-federal government use of the radio spectrum (including radio and television broadcasting), all interstate telecommunications (wire, satellite, and cable), and international communications that originate or terminate in the United States.
- Licensing: It is responsible for licensing radio and television stations, and ensuring compliance with the relevant regulations.
- Promoting Competition: The FCC works to promote competition, innovation, and investment in broadband services and facilities.
- Spectrum Management: One of its crucial roles is managing the nation’s airwaves, including spectrum allocation and assignment for various uses.
- Consumer Protection: The agency also enforces laws to protect consumers against fraud, unfair practices, and monopolistic behavior in the communications realm.
- Policy Making: The FCC develops policy concerning issues such as media ownership, net neutrality, privacy, and others that impact the nation’s communications.
- Emergency Communications: It plays a significant role in ensuring the reliability and security of critical communications infrastructure, particularly during emergencies.
- Digital Transition: The FCC has been instrumental in overseeing the transition from analog to digital broadcasting and the development and deployment of new communication technologies like 5G.
The FCC’s actions are watched closely by various stakeholders due to their far-reaching impact on how Americans communicate and access information.
The International Telecommunication Union (ITU) is a specialized agency of the United Nations responsible for issues that concern information and communication technologies. Established in 1865, originally as the International Telegraph Union, the ITU is one of the oldest international organizations. It plays a pivotal role in facilitating global communications and technology standards. Key aspects of the ITU include:
- Standardization: The ITU is responsible for developing international standards (ITU-T Recommendations) that facilitate seamless global telecommunications and ensure interoperable and efficient communication systems.
- Radio Spectrum Allocation: The ITU coordinates the global use of the radio spectrum (ITU-R Recommendations) and satellite orbits, ensuring non-interference and efficient use of these resources.
- Improving Access to ICTs: The organization works to improve access to information and communication technologies (ICTs) in underserved communities worldwide, promoting sustainable development.
- Regulatory Framework and Policies: ITU assists in developing regulatory frameworks and offers policy advice to ensure fair and equitable access to ICT services.
- Telecommunication Development: The ITU-D sector focuses on fostering international cooperation and solidarity in the delivery of technical assistance and the implementation of telecommunication/ICT projects in developing countries.
- Global Conferences and Exhibitions: ITU organizes the World Radiocommunication Conference (WRC), World Telecommunication Standardization Assembly (WTSA), and other significant events that shape the future of ICTs.
- Membership: ITU’s membership includes 193 Member States as well as over 800 private-sector entities, academic institutions, and international and regional organizations.
The ITU plays a crucial role in shaping global telecommunications’s technological and regulatory landscape, making it a cornerstone entity in modern communication and information exchange.
WIreless Technologies (20)
The evolution of Radio Access Network (RAN) solutions from 2G to 5G represents a journey of significant technological advancements, each generation introducing new capabilities and features. Here is an overview of how RAN solutions have evolved:
- 2G (GSM) RAN:
- Introduced in the 1990s, 2G was the first generation of digital cellular technology.
- It primarily focused on voice services and simple data transmission using technologies like GSM (Global System for Mobile Communications).
- 2G RANs utilized narrowband TDMA (Time Division Multiple Access).
- 3G RAN:
- Launched in the early 2000s, 3G brought higher data rates, enabling mobile internet access and improved voice call quality.
- Technologies like UMTS (Universal Mobile Telecommunications System) and later HSPA (High-Speed Packet Access) were used.
- 3G RANs used wideband CDMA (Code Division Multiple Access) for more efficient spectrum utilization.
- 4G (LTE) RAN:
- 4G, introduced in the late 2000s, marked a significant leap with LTE (Long-Term Evolution) technology, offering high-speed mobile broadband.
- LTE RANs provided much higher data rates, lower latency, and improved capacity compared to 3G.
- The focus shifted towards all-IP (Internet Protocol) based networks, enabling seamless internet and multimedia services.
- 5G RAN:
- 5G, rolling out since 2019, introduces even higher data rates, ultra-low latency, and massive network capacity.
- It supports advanced applications like IoT, augmented reality, and autonomous vehicles.
- 5G RANs utilize technologies like Massive MIMO (Multiple Input Multiple Output), beamforming, and network slicing.
- They operate across a broader range of frequencies, including sub-6 GHz and mmWave bands.
Each generation’s RAN has been characterized by advancements in data rate, efficiency, and the types of services it could support. From basic voice and text in 2G to multimedia and high-speed data in 4G, and now to a fully connected world with 5G, the RAN technology has continuously evolved to meet the growing demands of connectivity and innovation.
Radio Access Network (RAN) solutions are integral components of mobile telecommunications networks. They encompass the technology and infrastructure responsible for connecting mobile devices to the core network and managing wireless communication. Key aspects of RAN solutions include:
- Connection Handling: RAN solutions handle all radio connections between user devices (like smartphones and tablets) and the network, facilitating communication with the core network.
- Base Stations and Antennas: They comprise base stations (also known as cell sites) and antennas that cover specific geographic areas, known as cells. These base stations manage radio communication with devices in their coverage area.
- Types of RAN:
- Traditional RAN: In traditional RAN setups, hardware and software are typically proprietary and supplied by a single vendor. The network elements are closely integrated.
- Open RAN: Open RAN architectures promote open interfaces and interoperability between different vendors’ equipment, allowing more flexibility and vendor diversity.
- Technology Evolution: RAN solutions have evolved from 2G to 5G, with each generation bringing advancements in speed, capacity, and efficiency. Current developments focus on 5G RAN, offering high-speed data transmission and low latency.
- Virtualization and Centralization: Modern RAN solutions are moving towards virtualization and centralization, where traditional hardware elements are replaced with software-defined solutions that can be centrally managed.
- Cloud RAN (C-RAN): An emerging approach where RAN functionalities are hosted in cloud data centers, leading to more efficient resource utilization and better network management.
- Support for Diverse Applications: RAN solutions support a wide range of applications, from voice calls and texting to high-speed internet access, streaming, and IoT connectivity.
RAN solutions are crucial for the functioning of mobile networks, serving as the link between end-users and the broader network infrastructure, and are continually evolving to meet the demands of new technologies and applications.
The frequency bands used in global telecommunications are varied and designated for specific purposes, including mobile communication, broadcasting, satellite communication, and more. Here’s an overview of some key frequency bands used in telecom:
- Low Frequency (LF) Bands (30 kHz to 300 kHz):
- Primarily used for AM radio broadcasting, maritime communication, and navigation.
- Medium Frequency (MF) Bands (300 kHz to 3 MHz):
- Used for AM radio broadcasting and aviation communication.
- High Frequency (HF) Bands (3 MHz to 30 MHz):
- Utilized for shortwave radio broadcasting, amateur radio, and maritime communication.
- Very High Frequency (VHF) Bands (30 MHz to 300 MHz):
- Include FM radio broadcasting (88 MHz to 108 MHz) and VHF TV broadcasting.
- Used in aviation and maritime communication, and two-way radios.
- Ultra High Frequency (UHF) Bands (300 MHz to 3 GHz):
- Cover TV broadcasting and mobile communication (LTE, GSM).
- Include the 2.4 GHz band used for Wi-Fi and Bluetooth.
- Super High Frequency (SHF) Bands (3 GHz to 30 GHz):
- Encompass parts of the spectrum used for newer 4G and 5G cellular networks.
- Include bands used for satellite communication and radar systems.
- Extremely High Frequency (EHF) Bands (30 GHz to 300 GHz):
- Used in high-capacity wireless communication, millimeter-wave radar, and scientific research.
- 5G networks utilize some of these higher frequencies (e.g., around 28 GHz and 39 GHz) for mmWave communication.
- Cellular Frequency Bands:
- GSM Bands: 900 MHz and 1800 MHz in most parts of the world, 850 MHz and 1900 MHz in the Americas.
- 3G/UMTS Bands: 2100 MHz (Band 1) is the most widely used globally.
- 4G/LTE Bands: Numerous bands including 700 MHz, 800 MHz, 1800 MHz, 2600 MHz, and others.
- 5G Bands: Ranging from sub-1 GHz low bands to mid-band (3.5 GHz) and high-band mmWave frequencies.
The allocation of these bands can vary by region, and they are regulated by international organizations like the International Telecommunication Union (ITU) and national regulatory bodies such as the FCC in the United States or Ofcom in the United Kingdom.
A Radio Intelligent Controller (RIC) is a key component in modern wireless network architectures, particularly in Open Radio Access Networks (Open RAN or O-RAN). The RIC plays a critical role in optimizing and managing radio network functions through advanced algorithms and machine learning. Key aspects of a Radio Intelligent Controller include:
- Network Optimization: The RIC uses real-time analytics to optimize network performance, including managing resources, balancing loads, and enhancing connectivity.
- Automation and Intelligence: By incorporating artificial intelligence and machine learning, the RIC automates many network operations, improving efficiency and reducing the need for manual intervention.
- Open RAN Integration: In the context of Open RAN, the RIC is crucial for enabling interoperability and flexibility, allowing components from different vendors to work seamlessly together.
- Two Types of RICs:
- Near-Real-Time RIC: Focuses on optimizing network performance in a timescale of milliseconds to seconds. It manages functions like handovers, beamforming, and load balancing.
- Non-Real-Time RIC: Operates on a longer timescale (seconds to minutes) and is involved in broader network management functions like policy control, network slicing, and predictive analysis.
- Standardization and Open Interfaces: The development of RICs is guided by standardization bodies like the O-RAN Alliance, which promotes open interfaces and standardized software to foster innovation and vendor diversity.
- Enhanced User Experience: By optimizing network performance, RICs contribute to an enhanced user experience, offering better connectivity, reduced latency, and more reliable service.
- Scalability and Flexibility: RICs enable networks to scale more efficiently and adapt to changing demands, supporting the rollout of new services and technologies like 5G.
The RIC represents a significant evolution in radio network management, bringing intelligence and flexibility to the forefront of wireless network operations.
Adaptive Data Rate (ADR) is a feature in some wireless communication protocols, notably in LoRaWAN (Long Range Wide Area Network), which is part of the broader category of Low Power Wide Area Networks (LPWAN). ADR optimizes the data transmission rate, power consumption, and airtime of devices based on network conditions, device power capacity, and the quality of the radio link. Here’s how ADR functions and its importance:
- Optimization of Data Rate: ADR dynamically adjusts the data rate at which a device transmits. This is done by changing the spreading factor, bandwidth, and coding rate. Higher data rates can be used when the device is near a gateway, while lower data rates are used as the device moves further away.
- Power Efficiency: By adjusting the data rate, ADR also helps in conserving the battery life of devices. Devices that are closer to a gateway and can transmit at higher data rates will use less power, thus preserving battery life.
- Network Capacity Management: ADR helps in managing the capacity of the network. By ensuring that devices use the optimal data rate, it reduces the time on air for each transmission. This efficiency is crucial in LPWANs as it increases the overall capacity of the network to handle more devices.
- Adaptation to Changing Conditions: ADR responds to changing environmental conditions or changes in the location of the device. If a device’s transmissions start failing, ADR can lower the data rate to increase the chance of successful transmission.
- Manual Override: In some systems, ADR can be manually overridden. This is useful in scenarios where the network administrator knows the environment and can set the data rate to a fixed value for optimal performance.
- Use in LoRaWAN: In LoRaWAN, ADR is a critical feature, especially considering the varying distances between end devices and gateways and the need for long battery life in IoT applications.
- Limitations: ADR is not always suitable for devices that are mobile or experience rapidly changing RF conditions, as it may not react quickly enough to these changes.
In summary, Adaptive Data Rate is a key feature in wireless communication protocols like LoRaWAN, enhancing network efficiency, power consumption, and overall performance of the connected devices. It is particularly important in scenarios where devices must operate over extended periods on limited power sources, such as in many IoT applications.
GSM (Global System for Mobile Communications) is a standard developed by the European Telecommunications Standards Institute (ETSI) to describe the protocols for second-generation (2G) digital cellular networks used by mobile devices such as phones and tablets. Introduced in the 1990s, GSM was a major leap in mobile communication technology. Key aspects of GSM include:
- Digital Communication: GSM marked the transition from analog first-generation (1G) networks to digital, significantly improving voice quality, security, and capacity.
- Global Standard: As its name suggests, GSM became a global standard for mobile communication, facilitating international roaming and compatibility.
- Network Components: GSM networks consist of key subsystems like the Base Station Subsystem (BSS), Network and Switching Subsystem (NSS), and the Operations and Support Subsystem (OSS).
- SIM Cards: GSM introduced the use of SIM (Subscriber Identity Module) cards, which store subscriber data and facilitate mobile device identification and authentication on the network.
- Data Services: Besides voice communication, GSM supports data services such as SMS (Short Message Service) and later, GPRS (General Packet Radio Services) for basic internet connectivity.
- Encryption and Security: GSM networks employ encryption to secure voice and data communication, enhancing privacy and security.
- Frequency Bands: GSM operates in multiple frequency bands, like 900 MHz and 1800 MHz in Europe and 850 MHz and 1900 MHz in the Americas, catering to different regional requirements.
GSM set the foundation for modern mobile communication and led to the development of more advanced technologies like 3G (UMTS) and 4G (LTE).
Wi-Fi HaLow, designated as 802.11ah, is a wireless networking protocol developed by the Wi-Fi Alliance. It’s a part of the IEEE 802.11 set of WLAN standards, but it differs significantly from most of its predecessors. Here are some key aspects of Wi-Fi HaLow:
- Frequency Band: Wi-Fi HaLow operates in the sub-1 GHz spectrum, specifically in the 900 MHz band. This is a lower frequency compared to the 2.4 GHz and 5 GHz bands used by most Wi-Fi technologies. The lower frequency allows for better range and material penetration.
- Range and Coverage: One of the most significant benefits of Wi-Fi HaLow is its extended range. It can cover roughly double the distance of conventional Wi-Fi, making it ideal for reaching into areas that were previously difficult to cover.
- Penetration: The lower frequency also allows for better penetration through obstacles like walls and floors, making Wi-Fi HaLow more reliable in challenging environments.
- Power Efficiency: Wi-Fi HaLow is designed to be more power-efficient, which is crucial for Internet of Things (IoT) devices that often run on batteries. This efficiency extends the battery life of connected devices.
- IoT Applications: Due to its range, penetration, and power efficiency, Wi-Fi HaLow is particularly well-suited for IoT applications, especially in scenarios where devices need to be connected over larger areas or in challenging environments, like smart homes, agricultural settings, industrial sites, and smart cities.
- Device Connectivity: It supports a larger number of connected devices over a single access point compared to traditional Wi-Fi, which is beneficial for IoT environments where many devices need to be connected.
- Security and IP Support: Wi-Fi HaLow retains the high levels of security and native IP support that are characteristic of traditional Wi-Fi standards.
In summary, Wi-Fi HaLow extends the benefits of Wi-Fi to IoT applications, offering solutions to the unique challenges posed by the need for long-range, low-power, high-penetration wireless connectivity. It’s particularly relevant as the number of IoT devices continues to grow, requiring new solutions for connectivity.
LoRaWAN (Long Range Wide Area Network) is a protocol for low-power wide-area networks (LPWANs), designed to wirelessly connect battery-operated ‘things’ to the internet in regional, national, or global networks. It’s particularly useful for the Internet of Things (IoT) applications. Here are some key characteristics and aspects of LoRaWAN:
- Long Range Communication: LoRaWAN is known for its long-range capabilities, often reaching several kilometers in rural areas and penetrating dense urban or indoor environments.
- Low Power Consumption: Devices using LoRaWAN are designed to be power-efficient, which is critical for IoT applications where devices often run on batteries and need to operate for extended periods without maintenance.
- Secure Communication: LoRaWAN includes end-to-end encryption, ensuring secure data transmission, which is crucial in many IoT applications.
- Low Bandwidth: LoRaWAN is optimized for low data rate applications. It’s not suitable for large amounts of data or high-speed communication but is ideal for applications that only need to send small amounts of data over long intervals.
- Star-of-Stars Network Topology: In LoRaWAN networks, gateways relay messages between end-devices and a central network server. The gateways are connected to the network server via standard IP connections, while end-devices use single-hop wireless communication to one or many gateways.
- Adaptive Data Rate (ADR): LoRaWAN can optimize data rates and RF output to balance power consumption, airtime, and network capacity.
- Applications: It’s used in a variety of applications, including smart meters, smart agriculture, smart cities, and environmental monitoring.
- Network Architecture: The architecture is typically laid out in a hierarchical topology to enhance scalability and battery life for end-devices.
- License-Free Frequency Band: LoRaWAN operates in license-free bands such as the industrial, scientific, and medical (ISM) radio bands.
LoRaWAN is an essential technology for IoT ecosystems, especially in scenarios where devices need to communicate over long distances, consume minimal power, and send small amounts of data.
Low Power Wide Area Networks (LPWAN) are a type of wireless telecommunication network designed to allow long-range communications at a low bit rate among connected devices, typically used for M2M (Machine to Machine) and IoT (Internet of Things) applications. Key characteristics and advantages of LPWAN include:
- Long Range: LPWAN technologies are designed to provide wide-area coverage, often covering a radius of several kilometers, even in challenging environments such as urban or industrial areas.
- Low Power Consumption: Devices connected via LPWAN are optimized for low power consumption, which allows them to operate for years on a small battery. This is crucial for IoT applications where devices are often deployed in locations where regular maintenance or battery replacement is not feasible.
- Low Data Rate: LPWAN is optimized for transmissions that require a low data rate. It’s ideal for applications that only need to send small amounts of data intermittently, rather than streaming large quantities of data continuously.
- Cost-Effectiveness: The infrastructure and device costs associated with LPWAN are generally lower compared to other types of wireless networks. This makes LPWAN a practical choice for a wide range of IoT applications.
- Applications: LPWAN is used in a variety of applications, including smart meters, smart agriculture, asset tracking, and environmental monitoring.
- Examples of LPWAN Technologies: Some of the well-known LPWAN technologies include LoRaWAN (Long Range Wide Area Network), NB-IoT (Narrowband IoT), and Sigfox.
In summary, LPWANs play a crucial role in the growth of IoT by connecting devices over long distances with minimal power consumption and lower costs, making it feasible to deploy large networks of sensors and devices.
MIMO (Multiple Input Multiple Output) is a wireless technology used in communication systems, particularly in modern Wi-Fi and cellular networks like LTE and 5G. It involves the use of multiple antennas at both the transmitter and receiver to improve communication performance. Key aspects of MIMO include:
- Increased Data Throughput: By using multiple antennas, MIMO can transmit more data simultaneously compared to systems with a single antenna, significantly increasing the network’s data throughput.
- Spatial Multiplexing: This technique, used in MIMO systems, transmits different data streams simultaneously over the same frequency band but through different spatial paths. It effectively multiplies the capacity of the radio channel.
- Diversity Gain: MIMO can provide diversity gain by transmitting the same data across different antennas, reducing the likelihood of data loss due to fading or interference.
- Improved Signal Quality: MIMO systems can improve signal quality and reduce error rates by combining multiple received signals, which have traveled through different paths and thus experienced different levels of fading and interference.
- Beamforming: Advanced MIMO systems use beamforming to direct the signal towards the intended receiver, enhancing the signal strength and reducing interference to and from other devices.
- Types of MIMO:
- SU-MIMO (Single-User MIMO): Involves one transmitter and one receiver, each with multiple antennas.
- MU-MIMO (Multi-User MIMO): Allows communication with multiple users simultaneously, each with one or more antennas.
- Applications: MIMO technology is a foundational element in modern wireless communication standards, including Wi-Fi (802.11n, ac, ax), LTE, and 5G networks.
MIMO technology represents a significant advancement in wireless communications, enabling more efficient and reliable transmission of data, and is essential for achieving the high-speed and high-capacity requirements of current and future wireless networks.
Open RAN (Open Radio Access Network) is an initiative to create more open and interoperable wireless network architectures. It represents a shift from traditional RAN solutions, which often involve proprietary, integrated systems from a single vendor, to a more modular and flexible approach. Key aspects of Open RAN include:
- Open Interfaces: Open RAN emphasizes the use of standardized, open interfaces between various components of the radio access network. This allows for interoperability between different vendors’ equipment.
- Decoupling Hardware and Software: It enables the decoupling of hardware and software functionalities in the network, allowing operators to mix and match hardware and software from different suppliers.
- Vendor Diversity and Innovation: By promoting open standards and interfaces, Open RAN encourages more vendors to participate in the ecosystem, fostering innovation and potentially reducing costs.
- Virtualization and Software-Defined Networking: Open RAN leverages virtualization technologies and software-defined networking (SDN) principles, leading to more flexible and scalable networks.
- Increased Efficiency and Agility: Networks based on Open RAN can adapt more quickly to changing demands and technologies, improving efficiency and service quality.
- Support for 5G and Beyond: Open RAN is seen as a key enabler for the rollout of 5G networks, offering the agility and scalability needed to support 5G’s diverse use cases.
- Organizations and Alliances: Several industry alliances and organizations, such as the O-RAN Alliance and the Telecom Infra Project (TIP), are driving the development and adoption of Open RAN standards.
Open RAN represents a transformative approach in the deployment and operation of mobile networks, promising to enhance competition, innovation, and flexibility in the telecom industry.
Quadrature Amplitude Modulation (QAM) is a modulation technique used in various forms of communication systems, including digital television and wireless communications. It combines two amplitude-modulated signals into a single channel, thereby increasing the bandwidth efficiency. Here’s a more detailed look at QAM:
- Combining Amplitude and Phase Modulation: QAM works by varying both the amplitude and the phase of a carrier signal. Essentially, it’s a blend of both amplitude modulation (AM) and phase modulation (PM).
- Constellation Diagram: In QAM, data points represented in a modulation are often visualized using a constellation diagram, which plots the amplitude and phase variations as points on a two-dimensional graph. Each point on the diagram represents a different symbol.
- Increased Data Rates: By varying both amplitude and phase, QAM can transmit more data per symbol compared to using either modulation technique alone. This makes it more bandwidth-efficient and enables higher data transmission rates.
- Applications in Digital Transmission: QAM is widely used in digital radio and television broadcasting, cable TV systems, and in some wireless communication systems like Wi-Fi and cellular networks.
- Variants of QAM: There are several variants of QAM, like 16-QAM, 64-QAM, 256-QAM, and others. The number denotes how many different symbols can be represented; for instance, 256-QAM can represent 256 different symbols. Higher QAM levels can transmit more bits per symbol, but they also require a higher signal-to-noise ratio to avoid errors.
- Adaptive QAM: In some communication systems, QAM can be adaptively changed depending on the channel conditions. For example, a system might use a higher level of QAM when signal conditions are good and a lower level when they are less favorable to maintain the quality of the transmission.
- Challenges with Higher QAM Levels: As the level of QAM increases, the spacing between constellation points becomes tighter, making the system more susceptible to noise and errors. Hence, higher QAM levels require better quality transmission channels.
In summary, QAM is a fundamental modulation technique that enables efficient use of available bandwidth by combining amplitude and phase modulation, widely used in modern digital communication systems.
Spectrum licensing refers to the regulatory process whereby national governments or regulatory bodies authorize the use of specific parts of the radio frequency spectrum by individuals, companies, or organizations. This process is crucial for managing the radio spectrum, which is a finite resource. Key aspects of spectrum licensing include:
- Regulatory Authority Involvement: Spectrum licensing is typically overseen by a national regulatory authority, such as the Federal Communications Commission (FCC) in the United States or Ofcom in the United Kingdom.
- Allocation and Assignment: The process involves allocating frequency bands for specific uses (such as mobile communication, broadcasting, or satellite transmission) and assigning specific frequencies or bands to licensees.
- License Types: There are various types of spectrum licenses, including exclusive use licenses, shared use licenses, and unlicensed spectrum allocations (like the bands used for Wi-Fi).
- Auctioning Spectrum: Many countries use auctions to allocate spectrum licenses, allowing companies to bid on the rights to use certain frequency bands. This method is often used for commercial purposes like mobile networks.
- Licensing Fees: Licensees typically pay a fee for spectrum use rights. Fees can vary based on the spectrum band, the geographic coverage of the license, and the duration of the license.
- Conditions and Regulations: Spectrum licenses come with conditions and regulations to ensure efficient and non-interfering use of the spectrum, including technical specifications, usage limitations, and compliance with international agreements.
- Spectrum Management: Effective spectrum licensing is a critical aspect of spectrum management, ensuring that this valuable resource is used efficiently and in a way that minimizes interference between different users.
- Economic and Strategic Importance: Spectrum licensing is not only a regulatory process but also of significant economic and strategic importance, influencing the development and deployment of wireless communication technologies.
Spectrum licensing is a key tool in the management of radio frequencies, balancing the need for efficient use of the spectrum, technological innovation, and economic considerations.
Spectrum Reallocation Strategy refers to the process of reassigning and repurposing frequency bands for different uses, typically within the context of wireless communications. This strategy is crucial in managing the finite resource of the radio spectrum, especially with the increasing demand for wireless services. Key aspects of Spectrum Reallocation Strategy include:
- Addressing Spectrum Scarcity: With the growing number of wireless devices and services, such as mobile phones, IoT devices, and broadband services, the demand for radio spectrum has significantly increased, leading to the need for efficient spectrum management.
- Reallocating for New Technologies: As new technologies like 5G emerge, reallocating spectrum bands to accommodate these technologies becomes necessary to ensure they have the necessary bandwidth to operate effectively.
- Balancing Interests: The process involves balancing the needs and interests of various stakeholders, including government agencies, private sector companies, and the public.
- Regulatory Decisions: National and international regulatory bodies, such as the Federal Communications Commission (FCC) in the U.S. or the International Telecommunication Union (ITU) globally, play a key role in making decisions about spectrum reallocation.
- Auctioning Spectrum: Often, reallocated spectrum is auctioned off to the highest bidder, providing a transparent and market-driven mechanism for allocation.
- Minimizing Disruption: Careful planning is required to minimize disruption to existing services and users in bands that are being reallocated.
- Economic Implications: Spectrum reallocation can have significant economic implications, both in terms of the revenue generated from spectrum auctions and the economic benefits of new technologies and services that use the reallocated spectrum.
Spectrum Reallocation Strategy is a critical aspect of modern telecommunications policy, ensuring that this valuable resource is used effectively to meet current and future needs.
A “License-Free Frequency Band” refers to parts of the radio spectrum that can be used without the need to acquire a license from regulatory authorities. These bands are open for public use under certain regulations and guidelines set by the governing bodies, like the Federal Communications Commission (FCC) in the United States or similar organizations in other countries. Key features and implications of these bands include:
- Open Access: Individuals and companies can operate devices in these bands without needing to obtain a license, which lowers the barriers to entry for developing and deploying wireless technologies.
- Regulatory Guidelines: Although no license is required, there are still regulations that govern the use of these bands. These typically include limits on transmission power, requirements for equipment to tolerate interference, and rules to minimize the risk of devices interfering with each other.
- Common Uses: License-free bands are commonly used for consumer wireless devices like Wi-Fi routers (in the 2.4 GHz and 5 GHz bands), Bluetooth devices, cordless telephones, and other short-range communication devices. They’re also used for industrial, scientific, and medical (ISM) applications.
- Popular License-Free Bands: The most well-known license-free bands are the ISM bands, which include frequencies around 900 MHz, 2.4 GHz, and 5 GHz. These bands are widely used for a variety of wireless communication technologies.
- Advantages: The primary advantage of using license-free bands is the reduced cost and complexity associated with bringing a wireless product to market. There’s no need to bid for spectrum rights or pay licensing fees.
- Challenges: A major challenge in these bands is the potential for interference, as many different devices and technologies may be operating in the same frequency range. This can impact the performance and reliability of wireless communications.
- Global Variation: The availability and specific regulations of license-free bands can vary from one country to another, so manufacturers need to ensure their devices comply with the regulations in each market where they are sold.
In summary, license-free frequency bands are crucial for a wide range of wireless technologies, especially for consumer and small-scale industrial applications. They enable easier access to the radio spectrum but come with the responsibility of adhering to regulations and managing interference.
Wi-Fi 4, officially known as IEEE 802.11n, is the fourth generation of Wi-Fi standards and was a significant advancement over the previous Wi-Fi standards, particularly IEEE 802.11g. Introduced in 2009, Wi-Fi 4 brought several key improvements to wireless networking:
- Increased Speed: Wi-Fi 4 offered higher maximum data rates, up to 600 Mbps under ideal conditions, which was a substantial improvement over the 54 Mbps maximum of its predecessor.
- MIMO Technology: Wi-Fi 4 introduced Multiple Input Multiple Output (MIMO) technology. This allowed the use of multiple antennas for both transmission and reception, enhancing data throughput and signal range.
- Dual-Band Operation: Wi-Fi 4 could operate on both the 2.4 GHz and 5 GHz bands, giving users the flexibility to choose the band with less interference and better performance.
- Wider Channel Bandwidth: It supported channel bandwidths of up to 40 MHz, wider than the 20 MHz channels of previous standards. This allowed for more data to be transmitted simultaneously.
- Improved Range and Reliability: The range and reliability of Wi-Fi connections were significantly improved, offering better performance at greater distances and in environments with physical obstructions.
- Backward Compatibility: Wi-Fi 4 was backward compatible with earlier Wi-Fi standards, ensuring that devices supporting older standards could still connect to Wi-Fi 4 networks.
Wi-Fi 4 played a crucial role in advancing wireless networking technology, facilitating faster speeds, increased range, and better overall performance, paving the way for the development of subsequent Wi-Fi generations.
Wi-Fi 5, known technically as IEEE 802.11ac, is the fifth generation of Wi-Fi standards. It was a significant improvement over its predecessor, Wi-Fi 4 (802.11n), and brought several advancements:
- Higher Data Rates: Wi-Fi 5 offers greater maximum data rates than Wi-Fi 4, primarily due to more efficient data encoding. This results in faster speeds for users.
- Dual-Band Operation: Unlike Wi-Fi 4, which primarily operates on the 2.4 GHz band, Wi-Fi 5 operates on both 2.4 GHz and 5 GHz bands. The 5 GHz band offers less interference and higher speeds.
- Wider Channel Bandwidth: Wi-Fi 5 supports wider channel bandwidths of up to 160 MHz (compared to the maximum of 40 MHz in Wi-Fi 4), allowing more data to be transmitted simultaneously.
- MU-MIMO (Multi-User, Multiple Input, Multiple Output): This technology enables a Wi-Fi router to communicate with multiple devices at the same time, increasing network efficiency and throughput.
- Beamforming: Beamforming technology in Wi-Fi 5 helps in focusing the Wi-Fi signal towards the device, rather than broadcasting it in all directions, which enhances the signal strength and reliability.
- Backward Compatibility: Wi-Fi 5 is backward compatible with previous Wi-Fi standards, ensuring that older devices can still connect to Wi-Fi 5 networks.
Wi-Fi 5 represented a substantial step forward in wireless networking technology, offering improved speeds, efficiency, and capacity, particularly for environments with high data demands.
Wi-Fi 6, officially known as IEEE 802.11ax, is the sixth generation of Wi-Fi standards and a significant upgrade over its predecessor, Wi-Fi 5 (802.11ac). Introduced to provide better performance in environments with a lot of connected devices, Wi-Fi 6 offers several improvements:
- Increased Data Rates: Wi-Fi 6 provides higher data rates compared to Wi-Fi 5, thanks to more efficient data encoding and larger channel bandwidth. This results in faster internet speeds and better performance.
- Improved Network Efficiency: One of the key features of Wi-Fi 6 is OFDMA (Orthogonal Frequency Division Multiple Access), which allows one transmission to deliver data to multiple devices at once. This significantly improves efficiency, especially in crowded networks.
- Better Performance in Congested Areas: Wi-Fi 6 shines in areas with many connected devices, such as stadiums, airports, and urban apartments. It reduces latency and improves throughput, making the network more responsive.
- Enhanced Battery Life for Connected Devices: Wi-Fi 6 introduces Target Wake Time (TWT), a feature that schedules communication between the router and devices. This reduces the amount of time devices need to keep their antennas active, conserving battery life.
- Improved Security: Wi-Fi 6 comes with WPA3, the latest Wi-Fi security protocol, which enhances user data protection, especially on public networks.
- Backward Compatibility: Wi-Fi 6 routers and devices are backward compatible with previous Wi-Fi standards, ensuring that older devices can still connect to new networks.
- Wider Channel Bandwidth: It supports 1024-QAM (Quadrature Amplitude Modulation), which increases throughput for emerging, bandwidth-intensive use cases.
- MU-MIMO Enhancements: Multi-user, multiple input, multiple output (MU-MIMO) technology allows more data to be transferred at once and enables an access point to communicate with more than one device simultaneously.
Wi-Fi 6 is designed for the next generation of connectivity, offering faster speeds, greater capacity, and better performance in environments with a lot of wireless devices.
Wi-Fi 7, technically known as 802.11be, is the forthcoming generation of Wi-Fi technology, following Wi-Fi 6 (802.11ax). It is being developed by the IEEE (Institute of Electrical and Electronics Engineers) and is expected to significantly enhance wireless networking performance. Key features and advancements of Wi-Fi 7 include:
- Higher Data Rates: Wi-Fi 7 is anticipated to offer substantially higher maximum data rates than Wi-Fi 6, potentially up to 30-40 Gbps. This is achieved through increased bandwidth and more efficient use of the wireless spectrum.
- Enhanced Bandwidth Utilization: The standard aims to support wider channel bandwidths, up to 320 MHz, and improve the utilization of available frequency bands, including 2.4 GHz, 5 GHz, and 6 GHz.
- Multi-Link Operation (MLO): A significant feature of Wi-Fi 7, MLO allows devices to use multiple bands and channels simultaneously. This can enhance throughput, reduce latency, and improve reliability.
- Advanced Modulation Techniques: Wi-Fi 7 is expected to support 4096-QAM (Quadrature Amplitude Modulation), enabling more bits to be transmitted with each signal, thus increasing the overall data throughput.
- Improved Latency: The new standard aims to significantly reduce latency, which is crucial for applications that require real-time communication, such as online gaming, augmented reality, and virtual reality.
- Increased Network Efficiency: Wi-Fi 7 is designed to be more efficient, especially in environments with many active devices, by utilizing more sophisticated technologies like improved spatial reuse and better scheduling algorithms.
- Backward Compatibility: Like its predecessors, Wi-Fi 7 is expected to be backward compatible with older Wi-Fi standards, ensuring a smooth transition for users upgrading their network hardware.
- Better Power Management: Features like Target Wake Time (TWT) are likely to be enhanced to improve power efficiency for IoT and mobile devices, extending their battery life.
Wi-Fi 7, with its advanced capabilities, is poised to meet the growing demands for higher data rates, lower latency, and more efficient networking in increasingly crowded and diverse wireless environments. As of my last update in April 2023, Wi-Fi 7 is still in the development phase, with its finalization and widespread adoption expected in the following years.
Wi-Fi HaLow, designated as 802.11ah, is a wireless networking protocol developed under the IEEE 802.11 standard. It’s specifically designed for the Internet of Things (IoT) applications. Key features and aspects of Wi-Fi HaLow include:
- Sub-GHz Operation: Unlike traditional Wi-Fi that operates in the 2.4 GHz and 5 GHz bands, Wi-Fi HaLow operates in frequency bands below 1 GHz. This allows for better range and penetration through obstacles like walls and floors.
- Extended Range: Wi-Fi HaLow is known for its long-range capabilities, typically offering coverage over several kilometers. This makes it ideal for IoT applications spread over large areas, like agricultural or industrial environments.
- Low Power Consumption: Devices using Wi-Fi HaLow are designed for low power usage, which is essential for IoT devices, many of which need to operate for years on a small battery.
- High Device Capacity: Wi-Fi HaLow can support thousands of connected devices under a single access point, much more than traditional Wi-Fi. This is particularly important for IoT applications, where many devices are often deployed in a condensed area.
- Use Cases: Wi-Fi HaLow is suited for a range of IoT applications, including smart home and building automation, agricultural and environmental sensors, and industrial monitoring.
- Compatibility and Security: Wi-Fi HaLow retains the core characteristics of the Wi-Fi protocol, including security protocols and ease of integration with existing Wi-Fi technologies.
- Data Rates: While it supports lower data rates compared to conventional Wi-Fi, it’s sufficient for the typical data needs of IoT devices, which usually transmit small amounts of data.
In summary, Wi-Fi HaLow extends the versatility of Wi-Fi to IoT applications, offering solutions for long-range, low-power, and high-density connectivity challenges.