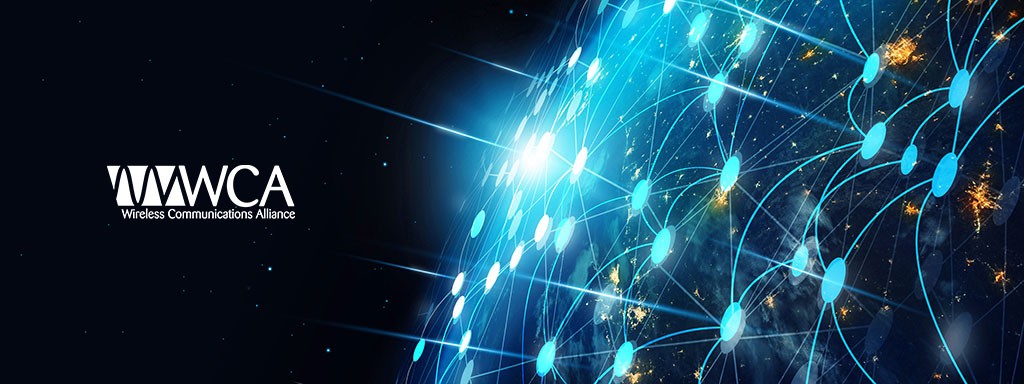
Wireless and Connected Technology Explained – We explore the complexities of connected technology. We provide insightful analyses, delve into current trends, and forecast future developments. Whether you are tech-savvy or simply curious, our journey through 5G, IoT, and network innovations will enlighten you. Welcome to our detailed examination of connected tech, where we explore 5G/6G, IIoT, Edge computing. Connected AI, mmWave, diverse network structures, and groundbreaking technologies are shaping our digital era.
Networks (1)
GSM (Global System for Mobile Communications) is a standard developed by the European Telecommunications Standards Institute (ETSI) to describe the protocols for second-generation (2G) digital cellular networks used by mobile devices such as phones and tablets. Introduced in the 1990s, GSM was a major leap in mobile communication technology. Key aspects of GSM include:
- Digital Communication: GSM marked the transition from analog first-generation (1G) networks to digital, significantly improving voice quality, security, and capacity.
- Global Standard: As its name suggests, GSM became a global standard for mobile communication, facilitating international roaming and compatibility.
- Network Components: GSM networks consist of key subsystems like the Base Station Subsystem (BSS), Network and Switching Subsystem (NSS), and the Operations and Support Subsystem (OSS).
- SIM Cards: GSM introduced the use of SIM (Subscriber Identity Module) cards, which store subscriber data and facilitate mobile device identification and authentication on the network.
- Data Services: Besides voice communication, GSM supports data services such as SMS (Short Message Service) and later, GPRS (General Packet Radio Services) for basic internet connectivity.
- Encryption and Security: GSM networks employ encryption to secure voice and data communication, enhancing privacy and security.
- Frequency Bands: GSM operates in multiple frequency bands, like 900 MHz and 1800 MHz in Europe and 850 MHz and 1900 MHz in the Americas, catering to different regional requirements.
GSM set the foundation for modern mobile communication and led to the development of more advanced technologies like 3G (UMTS) and 4G (LTE).
WIreless Technologies (20)
The evolution of Radio Access Network (RAN) solutions from 2G to 5G represents a journey of significant technological advancements, each generation introducing new capabilities and features. Here is an overview of how RAN solutions have evolved:
- 2G (GSM) RAN:
- Introduced in the 1990s, 2G was the first generation of digital cellular technology.
- It primarily focused on voice services and simple data transmission using technologies like GSM (Global System for Mobile Communications).
- 2G RANs utilized narrowband TDMA (Time Division Multiple Access).
- 3G RAN:
- Launched in the early 2000s, 3G brought higher data rates, enabling mobile internet access and improved voice call quality.
- Technologies like UMTS (Universal Mobile Telecommunications System) and later HSPA (High-Speed Packet Access) were used.
- 3G RANs used wideband CDMA (Code Division Multiple Access) for more efficient spectrum utilization.
- 4G (LTE) RAN:
- 4G, introduced in the late 2000s, marked a significant leap with LTE (Long-Term Evolution) technology, offering high-speed mobile broadband.
- LTE RANs provided much higher data rates, lower latency, and improved capacity compared to 3G.
- The focus shifted towards all-IP (Internet Protocol) based networks, enabling seamless internet and multimedia services.
- 5G RAN:
- 5G, rolling out since 2019, introduces even higher data rates, ultra-low latency, and massive network capacity.
- It supports advanced applications like IoT, augmented reality, and autonomous vehicles.
- 5G RANs utilize technologies like Massive MIMO (Multiple Input Multiple Output), beamforming, and network slicing.
- They operate across a broader range of frequencies, including sub-6 GHz and mmWave bands.
Each generation’s RAN has been characterized by advancements in data rate, efficiency, and the types of services it could support. From basic voice and text in 2G to multimedia and high-speed data in 4G, and now to a fully connected world with 5G, the RAN technology has continuously evolved to meet the growing demands of connectivity and innovation.
Radio Access Network (RAN) solutions are integral components of mobile telecommunications networks. They encompass the technology and infrastructure responsible for connecting mobile devices to the core network and managing wireless communication. Key aspects of RAN solutions include:
- Connection Handling: RAN solutions handle all radio connections between user devices (like smartphones and tablets) and the network, facilitating communication with the core network.
- Base Stations and Antennas: They comprise base stations (also known as cell sites) and antennas that cover specific geographic areas, known as cells. These base stations manage radio communication with devices in their coverage area.
- Types of RAN:
- Traditional RAN: In traditional RAN setups, hardware and software are typically proprietary and supplied by a single vendor. The network elements are closely integrated.
- Open RAN: Open RAN architectures promote open interfaces and interoperability between different vendors’ equipment, allowing more flexibility and vendor diversity.
- Technology Evolution: RAN solutions have evolved from 2G to 5G, with each generation bringing advancements in speed, capacity, and efficiency. Current developments focus on 5G RAN, offering high-speed data transmission and low latency.
- Virtualization and Centralization: Modern RAN solutions are moving towards virtualization and centralization, where traditional hardware elements are replaced with software-defined solutions that can be centrally managed.
- Cloud RAN (C-RAN): An emerging approach where RAN functionalities are hosted in cloud data centers, leading to more efficient resource utilization and better network management.
- Support for Diverse Applications: RAN solutions support a wide range of applications, from voice calls and texting to high-speed internet access, streaming, and IoT connectivity.
RAN solutions are crucial for the functioning of mobile networks, serving as the link between end-users and the broader network infrastructure, and are continually evolving to meet the demands of new technologies and applications.
The frequency bands used in global telecommunications are varied and designated for specific purposes, including mobile communication, broadcasting, satellite communication, and more. Here’s an overview of some key frequency bands used in telecom:
- Low Frequency (LF) Bands (30 kHz to 300 kHz):
- Primarily used for AM radio broadcasting, maritime communication, and navigation.
- Medium Frequency (MF) Bands (300 kHz to 3 MHz):
- Used for AM radio broadcasting and aviation communication.
- High Frequency (HF) Bands (3 MHz to 30 MHz):
- Utilized for shortwave radio broadcasting, amateur radio, and maritime communication.
- Very High Frequency (VHF) Bands (30 MHz to 300 MHz):
- Include FM radio broadcasting (88 MHz to 108 MHz) and VHF TV broadcasting.
- Used in aviation and maritime communication, and two-way radios.
- Ultra High Frequency (UHF) Bands (300 MHz to 3 GHz):
- Cover TV broadcasting and mobile communication (LTE, GSM).
- Include the 2.4 GHz band used for Wi-Fi and Bluetooth.
- Super High Frequency (SHF) Bands (3 GHz to 30 GHz):
- Encompass parts of the spectrum used for newer 4G and 5G cellular networks.
- Include bands used for satellite communication and radar systems.
- Extremely High Frequency (EHF) Bands (30 GHz to 300 GHz):
- Used in high-capacity wireless communication, millimeter-wave radar, and scientific research.
- 5G networks utilize some of these higher frequencies (e.g., around 28 GHz and 39 GHz) for mmWave communication.
- Cellular Frequency Bands:
- GSM Bands: 900 MHz and 1800 MHz in most parts of the world, 850 MHz and 1900 MHz in the Americas.
- 3G/UMTS Bands: 2100 MHz (Band 1) is the most widely used globally.
- 4G/LTE Bands: Numerous bands including 700 MHz, 800 MHz, 1800 MHz, 2600 MHz, and others.
- 5G Bands: Ranging from sub-1 GHz low bands to mid-band (3.5 GHz) and high-band mmWave frequencies.
The allocation of these bands can vary by region, and they are regulated by international organizations like the International Telecommunication Union (ITU) and national regulatory bodies such as the FCC in the United States or Ofcom in the United Kingdom.
A Radio Intelligent Controller (RIC) is a key component in modern wireless network architectures, particularly in Open Radio Access Networks (Open RAN or O-RAN). The RIC plays a critical role in optimizing and managing radio network functions through advanced algorithms and machine learning. Key aspects of a Radio Intelligent Controller include:
- Network Optimization: The RIC uses real-time analytics to optimize network performance, including managing resources, balancing loads, and enhancing connectivity.
- Automation and Intelligence: By incorporating artificial intelligence and machine learning, the RIC automates many network operations, improving efficiency and reducing the need for manual intervention.
- Open RAN Integration: In the context of Open RAN, the RIC is crucial for enabling interoperability and flexibility, allowing components from different vendors to work seamlessly together.
- Two Types of RICs:
- Near-Real-Time RIC: Focuses on optimizing network performance in a timescale of milliseconds to seconds. It manages functions like handovers, beamforming, and load balancing.
- Non-Real-Time RIC: Operates on a longer timescale (seconds to minutes) and is involved in broader network management functions like policy control, network slicing, and predictive analysis.
- Standardization and Open Interfaces: The development of RICs is guided by standardization bodies like the O-RAN Alliance, which promotes open interfaces and standardized software to foster innovation and vendor diversity.
- Enhanced User Experience: By optimizing network performance, RICs contribute to an enhanced user experience, offering better connectivity, reduced latency, and more reliable service.
- Scalability and Flexibility: RICs enable networks to scale more efficiently and adapt to changing demands, supporting the rollout of new services and technologies like 5G.
The RIC represents a significant evolution in radio network management, bringing intelligence and flexibility to the forefront of wireless network operations.
Adaptive Data Rate (ADR) is a feature in some wireless communication protocols, notably in LoRaWAN (Long Range Wide Area Network), which is part of the broader category of Low Power Wide Area Networks (LPWAN). ADR optimizes the data transmission rate, power consumption, and airtime of devices based on network conditions, device power capacity, and the quality of the radio link. Here’s how ADR functions and its importance:
- Optimization of Data Rate: ADR dynamically adjusts the data rate at which a device transmits. This is done by changing the spreading factor, bandwidth, and coding rate. Higher data rates can be used when the device is near a gateway, while lower data rates are used as the device moves further away.
- Power Efficiency: By adjusting the data rate, ADR also helps in conserving the battery life of devices. Devices that are closer to a gateway and can transmit at higher data rates will use less power, thus preserving battery life.
- Network Capacity Management: ADR helps in managing the capacity of the network. By ensuring that devices use the optimal data rate, it reduces the time on air for each transmission. This efficiency is crucial in LPWANs as it increases the overall capacity of the network to handle more devices.
- Adaptation to Changing Conditions: ADR responds to changing environmental conditions or changes in the location of the device. If a device’s transmissions start failing, ADR can lower the data rate to increase the chance of successful transmission.
- Manual Override: In some systems, ADR can be manually overridden. This is useful in scenarios where the network administrator knows the environment and can set the data rate to a fixed value for optimal performance.
- Use in LoRaWAN: In LoRaWAN, ADR is a critical feature, especially considering the varying distances between end devices and gateways and the need for long battery life in IoT applications.
- Limitations: ADR is not always suitable for devices that are mobile or experience rapidly changing RF conditions, as it may not react quickly enough to these changes.
In summary, Adaptive Data Rate is a key feature in wireless communication protocols like LoRaWAN, enhancing network efficiency, power consumption, and overall performance of the connected devices. It is particularly important in scenarios where devices must operate over extended periods on limited power sources, such as in many IoT applications.
GSM (Global System for Mobile Communications) is a standard developed by the European Telecommunications Standards Institute (ETSI) to describe the protocols for second-generation (2G) digital cellular networks used by mobile devices such as phones and tablets. Introduced in the 1990s, GSM was a major leap in mobile communication technology. Key aspects of GSM include:
- Digital Communication: GSM marked the transition from analog first-generation (1G) networks to digital, significantly improving voice quality, security, and capacity.
- Global Standard: As its name suggests, GSM became a global standard for mobile communication, facilitating international roaming and compatibility.
- Network Components: GSM networks consist of key subsystems like the Base Station Subsystem (BSS), Network and Switching Subsystem (NSS), and the Operations and Support Subsystem (OSS).
- SIM Cards: GSM introduced the use of SIM (Subscriber Identity Module) cards, which store subscriber data and facilitate mobile device identification and authentication on the network.
- Data Services: Besides voice communication, GSM supports data services such as SMS (Short Message Service) and later, GPRS (General Packet Radio Services) for basic internet connectivity.
- Encryption and Security: GSM networks employ encryption to secure voice and data communication, enhancing privacy and security.
- Frequency Bands: GSM operates in multiple frequency bands, like 900 MHz and 1800 MHz in Europe and 850 MHz and 1900 MHz in the Americas, catering to different regional requirements.
GSM set the foundation for modern mobile communication and led to the development of more advanced technologies like 3G (UMTS) and 4G (LTE).
Wi-Fi HaLow, designated as 802.11ah, is a wireless networking protocol developed by the Wi-Fi Alliance. It’s a part of the IEEE 802.11 set of WLAN standards, but it differs significantly from most of its predecessors. Here are some key aspects of Wi-Fi HaLow:
- Frequency Band: Wi-Fi HaLow operates in the sub-1 GHz spectrum, specifically in the 900 MHz band. This is a lower frequency compared to the 2.4 GHz and 5 GHz bands used by most Wi-Fi technologies. The lower frequency allows for better range and material penetration.
- Range and Coverage: One of the most significant benefits of Wi-Fi HaLow is its extended range. It can cover roughly double the distance of conventional Wi-Fi, making it ideal for reaching into areas that were previously difficult to cover.
- Penetration: The lower frequency also allows for better penetration through obstacles like walls and floors, making Wi-Fi HaLow more reliable in challenging environments.
- Power Efficiency: Wi-Fi HaLow is designed to be more power-efficient, which is crucial for Internet of Things (IoT) devices that often run on batteries. This efficiency extends the battery life of connected devices.
- IoT Applications: Due to its range, penetration, and power efficiency, Wi-Fi HaLow is particularly well-suited for IoT applications, especially in scenarios where devices need to be connected over larger areas or in challenging environments, like smart homes, agricultural settings, industrial sites, and smart cities.
- Device Connectivity: It supports a larger number of connected devices over a single access point compared to traditional Wi-Fi, which is beneficial for IoT environments where many devices need to be connected.
- Security and IP Support: Wi-Fi HaLow retains the high levels of security and native IP support that are characteristic of traditional Wi-Fi standards.
In summary, Wi-Fi HaLow extends the benefits of Wi-Fi to IoT applications, offering solutions to the unique challenges posed by the need for long-range, low-power, high-penetration wireless connectivity. It’s particularly relevant as the number of IoT devices continues to grow, requiring new solutions for connectivity.
LoRaWAN (Long Range Wide Area Network) is a protocol for low-power wide-area networks (LPWANs), designed to wirelessly connect battery-operated ‘things’ to the internet in regional, national, or global networks. It’s particularly useful for the Internet of Things (IoT) applications. Here are some key characteristics and aspects of LoRaWAN:
- Long Range Communication: LoRaWAN is known for its long-range capabilities, often reaching several kilometers in rural areas and penetrating dense urban or indoor environments.
- Low Power Consumption: Devices using LoRaWAN are designed to be power-efficient, which is critical for IoT applications where devices often run on batteries and need to operate for extended periods without maintenance.
- Secure Communication: LoRaWAN includes end-to-end encryption, ensuring secure data transmission, which is crucial in many IoT applications.
- Low Bandwidth: LoRaWAN is optimized for low data rate applications. It’s not suitable for large amounts of data or high-speed communication but is ideal for applications that only need to send small amounts of data over long intervals.
- Star-of-Stars Network Topology: In LoRaWAN networks, gateways relay messages between end-devices and a central network server. The gateways are connected to the network server via standard IP connections, while end-devices use single-hop wireless communication to one or many gateways.
- Adaptive Data Rate (ADR): LoRaWAN can optimize data rates and RF output to balance power consumption, airtime, and network capacity.
- Applications: It’s used in a variety of applications, including smart meters, smart agriculture, smart cities, and environmental monitoring.
- Network Architecture: The architecture is typically laid out in a hierarchical topology to enhance scalability and battery life for end-devices.
- License-Free Frequency Band: LoRaWAN operates in license-free bands such as the industrial, scientific, and medical (ISM) radio bands.
LoRaWAN is an essential technology for IoT ecosystems, especially in scenarios where devices need to communicate over long distances, consume minimal power, and send small amounts of data.
Low Power Wide Area Networks (LPWAN) are a type of wireless telecommunication network designed to allow long-range communications at a low bit rate among connected devices, typically used for M2M (Machine to Machine) and IoT (Internet of Things) applications. Key characteristics and advantages of LPWAN include:
- Long Range: LPWAN technologies are designed to provide wide-area coverage, often covering a radius of several kilometers, even in challenging environments such as urban or industrial areas.
- Low Power Consumption: Devices connected via LPWAN are optimized for low power consumption, which allows them to operate for years on a small battery. This is crucial for IoT applications where devices are often deployed in locations where regular maintenance or battery replacement is not feasible.
- Low Data Rate: LPWAN is optimized for transmissions that require a low data rate. It’s ideal for applications that only need to send small amounts of data intermittently, rather than streaming large quantities of data continuously.
- Cost-Effectiveness: The infrastructure and device costs associated with LPWAN are generally lower compared to other types of wireless networks. This makes LPWAN a practical choice for a wide range of IoT applications.
- Applications: LPWAN is used in a variety of applications, including smart meters, smart agriculture, asset tracking, and environmental monitoring.
- Examples of LPWAN Technologies: Some of the well-known LPWAN technologies include LoRaWAN (Long Range Wide Area Network), NB-IoT (Narrowband IoT), and Sigfox.
In summary, LPWANs play a crucial role in the growth of IoT by connecting devices over long distances with minimal power consumption and lower costs, making it feasible to deploy large networks of sensors and devices.
MIMO (Multiple Input Multiple Output) is a wireless technology used in communication systems, particularly in modern Wi-Fi and cellular networks like LTE and 5G. It involves the use of multiple antennas at both the transmitter and receiver to improve communication performance. Key aspects of MIMO include:
- Increased Data Throughput: By using multiple antennas, MIMO can transmit more data simultaneously compared to systems with a single antenna, significantly increasing the network’s data throughput.
- Spatial Multiplexing: This technique, used in MIMO systems, transmits different data streams simultaneously over the same frequency band but through different spatial paths. It effectively multiplies the capacity of the radio channel.
- Diversity Gain: MIMO can provide diversity gain by transmitting the same data across different antennas, reducing the likelihood of data loss due to fading or interference.
- Improved Signal Quality: MIMO systems can improve signal quality and reduce error rates by combining multiple received signals, which have traveled through different paths and thus experienced different levels of fading and interference.
- Beamforming: Advanced MIMO systems use beamforming to direct the signal towards the intended receiver, enhancing the signal strength and reducing interference to and from other devices.
- Types of MIMO:
- SU-MIMO (Single-User MIMO): Involves one transmitter and one receiver, each with multiple antennas.
- MU-MIMO (Multi-User MIMO): Allows communication with multiple users simultaneously, each with one or more antennas.
- Applications: MIMO technology is a foundational element in modern wireless communication standards, including Wi-Fi (802.11n, ac, ax), LTE, and 5G networks.
MIMO technology represents a significant advancement in wireless communications, enabling more efficient and reliable transmission of data, and is essential for achieving the high-speed and high-capacity requirements of current and future wireless networks.
Open RAN (Open Radio Access Network) is an initiative to create more open and interoperable wireless network architectures. It represents a shift from traditional RAN solutions, which often involve proprietary, integrated systems from a single vendor, to a more modular and flexible approach. Key aspects of Open RAN include:
- Open Interfaces: Open RAN emphasizes the use of standardized, open interfaces between various components of the radio access network. This allows for interoperability between different vendors’ equipment.
- Decoupling Hardware and Software: It enables the decoupling of hardware and software functionalities in the network, allowing operators to mix and match hardware and software from different suppliers.
- Vendor Diversity and Innovation: By promoting open standards and interfaces, Open RAN encourages more vendors to participate in the ecosystem, fostering innovation and potentially reducing costs.
- Virtualization and Software-Defined Networking: Open RAN leverages virtualization technologies and software-defined networking (SDN) principles, leading to more flexible and scalable networks.
- Increased Efficiency and Agility: Networks based on Open RAN can adapt more quickly to changing demands and technologies, improving efficiency and service quality.
- Support for 5G and Beyond: Open RAN is seen as a key enabler for the rollout of 5G networks, offering the agility and scalability needed to support 5G’s diverse use cases.
- Organizations and Alliances: Several industry alliances and organizations, such as the O-RAN Alliance and the Telecom Infra Project (TIP), are driving the development and adoption of Open RAN standards.
Open RAN represents a transformative approach in the deployment and operation of mobile networks, promising to enhance competition, innovation, and flexibility in the telecom industry.
Quadrature Amplitude Modulation (QAM) is a modulation technique used in various forms of communication systems, including digital television and wireless communications. It combines two amplitude-modulated signals into a single channel, thereby increasing the bandwidth efficiency. Here’s a more detailed look at QAM:
- Combining Amplitude and Phase Modulation: QAM works by varying both the amplitude and the phase of a carrier signal. Essentially, it’s a blend of both amplitude modulation (AM) and phase modulation (PM).
- Constellation Diagram: In QAM, data points represented in a modulation are often visualized using a constellation diagram, which plots the amplitude and phase variations as points on a two-dimensional graph. Each point on the diagram represents a different symbol.
- Increased Data Rates: By varying both amplitude and phase, QAM can transmit more data per symbol compared to using either modulation technique alone. This makes it more bandwidth-efficient and enables higher data transmission rates.
- Applications in Digital Transmission: QAM is widely used in digital radio and television broadcasting, cable TV systems, and in some wireless communication systems like Wi-Fi and cellular networks.
- Variants of QAM: There are several variants of QAM, like 16-QAM, 64-QAM, 256-QAM, and others. The number denotes how many different symbols can be represented; for instance, 256-QAM can represent 256 different symbols. Higher QAM levels can transmit more bits per symbol, but they also require a higher signal-to-noise ratio to avoid errors.
- Adaptive QAM: In some communication systems, QAM can be adaptively changed depending on the channel conditions. For example, a system might use a higher level of QAM when signal conditions are good and a lower level when they are less favorable to maintain the quality of the transmission.
- Challenges with Higher QAM Levels: As the level of QAM increases, the spacing between constellation points becomes tighter, making the system more susceptible to noise and errors. Hence, higher QAM levels require better quality transmission channels.
In summary, QAM is a fundamental modulation technique that enables efficient use of available bandwidth by combining amplitude and phase modulation, widely used in modern digital communication systems.
Spectrum licensing refers to the regulatory process whereby national governments or regulatory bodies authorize the use of specific parts of the radio frequency spectrum by individuals, companies, or organizations. This process is crucial for managing the radio spectrum, which is a finite resource. Key aspects of spectrum licensing include:
- Regulatory Authority Involvement: Spectrum licensing is typically overseen by a national regulatory authority, such as the Federal Communications Commission (FCC) in the United States or Ofcom in the United Kingdom.
- Allocation and Assignment: The process involves allocating frequency bands for specific uses (such as mobile communication, broadcasting, or satellite transmission) and assigning specific frequencies or bands to licensees.
- License Types: There are various types of spectrum licenses, including exclusive use licenses, shared use licenses, and unlicensed spectrum allocations (like the bands used for Wi-Fi).
- Auctioning Spectrum: Many countries use auctions to allocate spectrum licenses, allowing companies to bid on the rights to use certain frequency bands. This method is often used for commercial purposes like mobile networks.
- Licensing Fees: Licensees typically pay a fee for spectrum use rights. Fees can vary based on the spectrum band, the geographic coverage of the license, and the duration of the license.
- Conditions and Regulations: Spectrum licenses come with conditions and regulations to ensure efficient and non-interfering use of the spectrum, including technical specifications, usage limitations, and compliance with international agreements.
- Spectrum Management: Effective spectrum licensing is a critical aspect of spectrum management, ensuring that this valuable resource is used efficiently and in a way that minimizes interference between different users.
- Economic and Strategic Importance: Spectrum licensing is not only a regulatory process but also of significant economic and strategic importance, influencing the development and deployment of wireless communication technologies.
Spectrum licensing is a key tool in the management of radio frequencies, balancing the need for efficient use of the spectrum, technological innovation, and economic considerations.
Spectrum Reallocation Strategy refers to the process of reassigning and repurposing frequency bands for different uses, typically within the context of wireless communications. This strategy is crucial in managing the finite resource of the radio spectrum, especially with the increasing demand for wireless services. Key aspects of Spectrum Reallocation Strategy include:
- Addressing Spectrum Scarcity: With the growing number of wireless devices and services, such as mobile phones, IoT devices, and broadband services, the demand for radio spectrum has significantly increased, leading to the need for efficient spectrum management.
- Reallocating for New Technologies: As new technologies like 5G emerge, reallocating spectrum bands to accommodate these technologies becomes necessary to ensure they have the necessary bandwidth to operate effectively.
- Balancing Interests: The process involves balancing the needs and interests of various stakeholders, including government agencies, private sector companies, and the public.
- Regulatory Decisions: National and international regulatory bodies, such as the Federal Communications Commission (FCC) in the U.S. or the International Telecommunication Union (ITU) globally, play a key role in making decisions about spectrum reallocation.
- Auctioning Spectrum: Often, reallocated spectrum is auctioned off to the highest bidder, providing a transparent and market-driven mechanism for allocation.
- Minimizing Disruption: Careful planning is required to minimize disruption to existing services and users in bands that are being reallocated.
- Economic Implications: Spectrum reallocation can have significant economic implications, both in terms of the revenue generated from spectrum auctions and the economic benefits of new technologies and services that use the reallocated spectrum.
Spectrum Reallocation Strategy is a critical aspect of modern telecommunications policy, ensuring that this valuable resource is used effectively to meet current and future needs.
A “License-Free Frequency Band” refers to parts of the radio spectrum that can be used without the need to acquire a license from regulatory authorities. These bands are open for public use under certain regulations and guidelines set by the governing bodies, like the Federal Communications Commission (FCC) in the United States or similar organizations in other countries. Key features and implications of these bands include:
- Open Access: Individuals and companies can operate devices in these bands without needing to obtain a license, which lowers the barriers to entry for developing and deploying wireless technologies.
- Regulatory Guidelines: Although no license is required, there are still regulations that govern the use of these bands. These typically include limits on transmission power, requirements for equipment to tolerate interference, and rules to minimize the risk of devices interfering with each other.
- Common Uses: License-free bands are commonly used for consumer wireless devices like Wi-Fi routers (in the 2.4 GHz and 5 GHz bands), Bluetooth devices, cordless telephones, and other short-range communication devices. They’re also used for industrial, scientific, and medical (ISM) applications.
- Popular License-Free Bands: The most well-known license-free bands are the ISM bands, which include frequencies around 900 MHz, 2.4 GHz, and 5 GHz. These bands are widely used for a variety of wireless communication technologies.
- Advantages: The primary advantage of using license-free bands is the reduced cost and complexity associated with bringing a wireless product to market. There’s no need to bid for spectrum rights or pay licensing fees.
- Challenges: A major challenge in these bands is the potential for interference, as many different devices and technologies may be operating in the same frequency range. This can impact the performance and reliability of wireless communications.
- Global Variation: The availability and specific regulations of license-free bands can vary from one country to another, so manufacturers need to ensure their devices comply with the regulations in each market where they are sold.
In summary, license-free frequency bands are crucial for a wide range of wireless technologies, especially for consumer and small-scale industrial applications. They enable easier access to the radio spectrum but come with the responsibility of adhering to regulations and managing interference.
Wi-Fi 4, officially known as IEEE 802.11n, is the fourth generation of Wi-Fi standards and was a significant advancement over the previous Wi-Fi standards, particularly IEEE 802.11g. Introduced in 2009, Wi-Fi 4 brought several key improvements to wireless networking:
- Increased Speed: Wi-Fi 4 offered higher maximum data rates, up to 600 Mbps under ideal conditions, which was a substantial improvement over the 54 Mbps maximum of its predecessor.
- MIMO Technology: Wi-Fi 4 introduced Multiple Input Multiple Output (MIMO) technology. This allowed the use of multiple antennas for both transmission and reception, enhancing data throughput and signal range.
- Dual-Band Operation: Wi-Fi 4 could operate on both the 2.4 GHz and 5 GHz bands, giving users the flexibility to choose the band with less interference and better performance.
- Wider Channel Bandwidth: It supported channel bandwidths of up to 40 MHz, wider than the 20 MHz channels of previous standards. This allowed for more data to be transmitted simultaneously.
- Improved Range and Reliability: The range and reliability of Wi-Fi connections were significantly improved, offering better performance at greater distances and in environments with physical obstructions.
- Backward Compatibility: Wi-Fi 4 was backward compatible with earlier Wi-Fi standards, ensuring that devices supporting older standards could still connect to Wi-Fi 4 networks.
Wi-Fi 4 played a crucial role in advancing wireless networking technology, facilitating faster speeds, increased range, and better overall performance, paving the way for the development of subsequent Wi-Fi generations.
Wi-Fi 5, known technically as IEEE 802.11ac, is the fifth generation of Wi-Fi standards. It was a significant improvement over its predecessor, Wi-Fi 4 (802.11n), and brought several advancements:
- Higher Data Rates: Wi-Fi 5 offers greater maximum data rates than Wi-Fi 4, primarily due to more efficient data encoding. This results in faster speeds for users.
- Dual-Band Operation: Unlike Wi-Fi 4, which primarily operates on the 2.4 GHz band, Wi-Fi 5 operates on both 2.4 GHz and 5 GHz bands. The 5 GHz band offers less interference and higher speeds.
- Wider Channel Bandwidth: Wi-Fi 5 supports wider channel bandwidths of up to 160 MHz (compared to the maximum of 40 MHz in Wi-Fi 4), allowing more data to be transmitted simultaneously.
- MU-MIMO (Multi-User, Multiple Input, Multiple Output): This technology enables a Wi-Fi router to communicate with multiple devices at the same time, increasing network efficiency and throughput.
- Beamforming: Beamforming technology in Wi-Fi 5 helps in focusing the Wi-Fi signal towards the device, rather than broadcasting it in all directions, which enhances the signal strength and reliability.
- Backward Compatibility: Wi-Fi 5 is backward compatible with previous Wi-Fi standards, ensuring that older devices can still connect to Wi-Fi 5 networks.
Wi-Fi 5 represented a substantial step forward in wireless networking technology, offering improved speeds, efficiency, and capacity, particularly for environments with high data demands.
Wi-Fi 6, officially known as IEEE 802.11ax, is the sixth generation of Wi-Fi standards and a significant upgrade over its predecessor, Wi-Fi 5 (802.11ac). Introduced to provide better performance in environments with a lot of connected devices, Wi-Fi 6 offers several improvements:
- Increased Data Rates: Wi-Fi 6 provides higher data rates compared to Wi-Fi 5, thanks to more efficient data encoding and larger channel bandwidth. This results in faster internet speeds and better performance.
- Improved Network Efficiency: One of the key features of Wi-Fi 6 is OFDMA (Orthogonal Frequency Division Multiple Access), which allows one transmission to deliver data to multiple devices at once. This significantly improves efficiency, especially in crowded networks.
- Better Performance in Congested Areas: Wi-Fi 6 shines in areas with many connected devices, such as stadiums, airports, and urban apartments. It reduces latency and improves throughput, making the network more responsive.
- Enhanced Battery Life for Connected Devices: Wi-Fi 6 introduces Target Wake Time (TWT), a feature that schedules communication between the router and devices. This reduces the amount of time devices need to keep their antennas active, conserving battery life.
- Improved Security: Wi-Fi 6 comes with WPA3, the latest Wi-Fi security protocol, which enhances user data protection, especially on public networks.
- Backward Compatibility: Wi-Fi 6 routers and devices are backward compatible with previous Wi-Fi standards, ensuring that older devices can still connect to new networks.
- Wider Channel Bandwidth: It supports 1024-QAM (Quadrature Amplitude Modulation), which increases throughput for emerging, bandwidth-intensive use cases.
- MU-MIMO Enhancements: Multi-user, multiple input, multiple output (MU-MIMO) technology allows more data to be transferred at once and enables an access point to communicate with more than one device simultaneously.
Wi-Fi 6 is designed for the next generation of connectivity, offering faster speeds, greater capacity, and better performance in environments with a lot of wireless devices.
Wi-Fi 7, technically known as 802.11be, is the forthcoming generation of Wi-Fi technology, following Wi-Fi 6 (802.11ax). It is being developed by the IEEE (Institute of Electrical and Electronics Engineers) and is expected to significantly enhance wireless networking performance. Key features and advancements of Wi-Fi 7 include:
- Higher Data Rates: Wi-Fi 7 is anticipated to offer substantially higher maximum data rates than Wi-Fi 6, potentially up to 30-40 Gbps. This is achieved through increased bandwidth and more efficient use of the wireless spectrum.
- Enhanced Bandwidth Utilization: The standard aims to support wider channel bandwidths, up to 320 MHz, and improve the utilization of available frequency bands, including 2.4 GHz, 5 GHz, and 6 GHz.
- Multi-Link Operation (MLO): A significant feature of Wi-Fi 7, MLO allows devices to use multiple bands and channels simultaneously. This can enhance throughput, reduce latency, and improve reliability.
- Advanced Modulation Techniques: Wi-Fi 7 is expected to support 4096-QAM (Quadrature Amplitude Modulation), enabling more bits to be transmitted with each signal, thus increasing the overall data throughput.
- Improved Latency: The new standard aims to significantly reduce latency, which is crucial for applications that require real-time communication, such as online gaming, augmented reality, and virtual reality.
- Increased Network Efficiency: Wi-Fi 7 is designed to be more efficient, especially in environments with many active devices, by utilizing more sophisticated technologies like improved spatial reuse and better scheduling algorithms.
- Backward Compatibility: Like its predecessors, Wi-Fi 7 is expected to be backward compatible with older Wi-Fi standards, ensuring a smooth transition for users upgrading their network hardware.
- Better Power Management: Features like Target Wake Time (TWT) are likely to be enhanced to improve power efficiency for IoT and mobile devices, extending their battery life.
Wi-Fi 7, with its advanced capabilities, is poised to meet the growing demands for higher data rates, lower latency, and more efficient networking in increasingly crowded and diverse wireless environments. As of my last update in April 2023, Wi-Fi 7 is still in the development phase, with its finalization and widespread adoption expected in the following years.
Wi-Fi HaLow, designated as 802.11ah, is a wireless networking protocol developed under the IEEE 802.11 standard. It’s specifically designed for the Internet of Things (IoT) applications. Key features and aspects of Wi-Fi HaLow include:
- Sub-GHz Operation: Unlike traditional Wi-Fi that operates in the 2.4 GHz and 5 GHz bands, Wi-Fi HaLow operates in frequency bands below 1 GHz. This allows for better range and penetration through obstacles like walls and floors.
- Extended Range: Wi-Fi HaLow is known for its long-range capabilities, typically offering coverage over several kilometers. This makes it ideal for IoT applications spread over large areas, like agricultural or industrial environments.
- Low Power Consumption: Devices using Wi-Fi HaLow are designed for low power usage, which is essential for IoT devices, many of which need to operate for years on a small battery.
- High Device Capacity: Wi-Fi HaLow can support thousands of connected devices under a single access point, much more than traditional Wi-Fi. This is particularly important for IoT applications, where many devices are often deployed in a condensed area.
- Use Cases: Wi-Fi HaLow is suited for a range of IoT applications, including smart home and building automation, agricultural and environmental sensors, and industrial monitoring.
- Compatibility and Security: Wi-Fi HaLow retains the core characteristics of the Wi-Fi protocol, including security protocols and ease of integration with existing Wi-Fi technologies.
- Data Rates: While it supports lower data rates compared to conventional Wi-Fi, it’s sufficient for the typical data needs of IoT devices, which usually transmit small amounts of data.
In summary, Wi-Fi HaLow extends the versatility of Wi-Fi to IoT applications, offering solutions for long-range, low-power, and high-density connectivity challenges.
Networks (1)
GSM (Global System for Mobile Communications) is a standard developed by the European Telecommunications Standards Institute (ETSI) to describe the protocols for second-generation (2G) digital cellular networks used by mobile devices such as phones and tablets. Introduced in the 1990s, GSM was a major leap in mobile communication technology. Key aspects of GSM include:
- Digital Communication: GSM marked the transition from analog first-generation (1G) networks to digital, significantly improving voice quality, security, and capacity.
- Global Standard: As its name suggests, GSM became a global standard for mobile communication, facilitating international roaming and compatibility.
- Network Components: GSM networks consist of key subsystems like the Base Station Subsystem (BSS), Network and Switching Subsystem (NSS), and the Operations and Support Subsystem (OSS).
- SIM Cards: GSM introduced the use of SIM (Subscriber Identity Module) cards, which store subscriber data and facilitate mobile device identification and authentication on the network.
- Data Services: Besides voice communication, GSM supports data services such as SMS (Short Message Service) and later, GPRS (General Packet Radio Services) for basic internet connectivity.
- Encryption and Security: GSM networks employ encryption to secure voice and data communication, enhancing privacy and security.
- Frequency Bands: GSM operates in multiple frequency bands, like 900 MHz and 1800 MHz in Europe and 850 MHz and 1900 MHz in the Americas, catering to different regional requirements.
GSM set the foundation for modern mobile communication and led to the development of more advanced technologies like 3G (UMTS) and 4G (LTE).
WIreless Technologies (20)
The evolution of Radio Access Network (RAN) solutions from 2G to 5G represents a journey of significant technological advancements, each generation introducing new capabilities and features. Here is an overview of how RAN solutions have evolved:
- 2G (GSM) RAN:
- Introduced in the 1990s, 2G was the first generation of digital cellular technology.
- It primarily focused on voice services and simple data transmission using technologies like GSM (Global System for Mobile Communications).
- 2G RANs utilized narrowband TDMA (Time Division Multiple Access).
- 3G RAN:
- Launched in the early 2000s, 3G brought higher data rates, enabling mobile internet access and improved voice call quality.
- Technologies like UMTS (Universal Mobile Telecommunications System) and later HSPA (High-Speed Packet Access) were used.
- 3G RANs used wideband CDMA (Code Division Multiple Access) for more efficient spectrum utilization.
- 4G (LTE) RAN:
- 4G, introduced in the late 2000s, marked a significant leap with LTE (Long-Term Evolution) technology, offering high-speed mobile broadband.
- LTE RANs provided much higher data rates, lower latency, and improved capacity compared to 3G.
- The focus shifted towards all-IP (Internet Protocol) based networks, enabling seamless internet and multimedia services.
- 5G RAN:
- 5G, rolling out since 2019, introduces even higher data rates, ultra-low latency, and massive network capacity.
- It supports advanced applications like IoT, augmented reality, and autonomous vehicles.
- 5G RANs utilize technologies like Massive MIMO (Multiple Input Multiple Output), beamforming, and network slicing.
- They operate across a broader range of frequencies, including sub-6 GHz and mmWave bands.
Each generation’s RAN has been characterized by advancements in data rate, efficiency, and the types of services it could support. From basic voice and text in 2G to multimedia and high-speed data in 4G, and now to a fully connected world with 5G, the RAN technology has continuously evolved to meet the growing demands of connectivity and innovation.
Radio Access Network (RAN) solutions are integral components of mobile telecommunications networks. They encompass the technology and infrastructure responsible for connecting mobile devices to the core network and managing wireless communication. Key aspects of RAN solutions include:
- Connection Handling: RAN solutions handle all radio connections between user devices (like smartphones and tablets) and the network, facilitating communication with the core network.
- Base Stations and Antennas: They comprise base stations (also known as cell sites) and antennas that cover specific geographic areas, known as cells. These base stations manage radio communication with devices in their coverage area.
- Types of RAN:
- Traditional RAN: In traditional RAN setups, hardware and software are typically proprietary and supplied by a single vendor. The network elements are closely integrated.
- Open RAN: Open RAN architectures promote open interfaces and interoperability between different vendors’ equipment, allowing more flexibility and vendor diversity.
- Technology Evolution: RAN solutions have evolved from 2G to 5G, with each generation bringing advancements in speed, capacity, and efficiency. Current developments focus on 5G RAN, offering high-speed data transmission and low latency.
- Virtualization and Centralization: Modern RAN solutions are moving towards virtualization and centralization, where traditional hardware elements are replaced with software-defined solutions that can be centrally managed.
- Cloud RAN (C-RAN): An emerging approach where RAN functionalities are hosted in cloud data centers, leading to more efficient resource utilization and better network management.
- Support for Diverse Applications: RAN solutions support a wide range of applications, from voice calls and texting to high-speed internet access, streaming, and IoT connectivity.
RAN solutions are crucial for the functioning of mobile networks, serving as the link between end-users and the broader network infrastructure, and are continually evolving to meet the demands of new technologies and applications.
The frequency bands used in global telecommunications are varied and designated for specific purposes, including mobile communication, broadcasting, satellite communication, and more. Here’s an overview of some key frequency bands used in telecom:
- Low Frequency (LF) Bands (30 kHz to 300 kHz):
- Primarily used for AM radio broadcasting, maritime communication, and navigation.
- Medium Frequency (MF) Bands (300 kHz to 3 MHz):
- Used for AM radio broadcasting and aviation communication.
- High Frequency (HF) Bands (3 MHz to 30 MHz):
- Utilized for shortwave radio broadcasting, amateur radio, and maritime communication.
- Very High Frequency (VHF) Bands (30 MHz to 300 MHz):
- Include FM radio broadcasting (88 MHz to 108 MHz) and VHF TV broadcasting.
- Used in aviation and maritime communication, and two-way radios.
- Ultra High Frequency (UHF) Bands (300 MHz to 3 GHz):
- Cover TV broadcasting and mobile communication (LTE, GSM).
- Include the 2.4 GHz band used for Wi-Fi and Bluetooth.
- Super High Frequency (SHF) Bands (3 GHz to 30 GHz):
- Encompass parts of the spectrum used for newer 4G and 5G cellular networks.
- Include bands used for satellite communication and radar systems.
- Extremely High Frequency (EHF) Bands (30 GHz to 300 GHz):
- Used in high-capacity wireless communication, millimeter-wave radar, and scientific research.
- 5G networks utilize some of these higher frequencies (e.g., around 28 GHz and 39 GHz) for mmWave communication.
- Cellular Frequency Bands:
- GSM Bands: 900 MHz and 1800 MHz in most parts of the world, 850 MHz and 1900 MHz in the Americas.
- 3G/UMTS Bands: 2100 MHz (Band 1) is the most widely used globally.
- 4G/LTE Bands: Numerous bands including 700 MHz, 800 MHz, 1800 MHz, 2600 MHz, and others.
- 5G Bands: Ranging from sub-1 GHz low bands to mid-band (3.5 GHz) and high-band mmWave frequencies.
The allocation of these bands can vary by region, and they are regulated by international organizations like the International Telecommunication Union (ITU) and national regulatory bodies such as the FCC in the United States or Ofcom in the United Kingdom.
A Radio Intelligent Controller (RIC) is a key component in modern wireless network architectures, particularly in Open Radio Access Networks (Open RAN or O-RAN). The RIC plays a critical role in optimizing and managing radio network functions through advanced algorithms and machine learning. Key aspects of a Radio Intelligent Controller include:
- Network Optimization: The RIC uses real-time analytics to optimize network performance, including managing resources, balancing loads, and enhancing connectivity.
- Automation and Intelligence: By incorporating artificial intelligence and machine learning, the RIC automates many network operations, improving efficiency and reducing the need for manual intervention.
- Open RAN Integration: In the context of Open RAN, the RIC is crucial for enabling interoperability and flexibility, allowing components from different vendors to work seamlessly together.
- Two Types of RICs:
- Near-Real-Time RIC: Focuses on optimizing network performance in a timescale of milliseconds to seconds. It manages functions like handovers, beamforming, and load balancing.
- Non-Real-Time RIC: Operates on a longer timescale (seconds to minutes) and is involved in broader network management functions like policy control, network slicing, and predictive analysis.
- Standardization and Open Interfaces: The development of RICs is guided by standardization bodies like the O-RAN Alliance, which promotes open interfaces and standardized software to foster innovation and vendor diversity.
- Enhanced User Experience: By optimizing network performance, RICs contribute to an enhanced user experience, offering better connectivity, reduced latency, and more reliable service.
- Scalability and Flexibility: RICs enable networks to scale more efficiently and adapt to changing demands, supporting the rollout of new services and technologies like 5G.
The RIC represents a significant evolution in radio network management, bringing intelligence and flexibility to the forefront of wireless network operations.
Adaptive Data Rate (ADR) is a feature in some wireless communication protocols, notably in LoRaWAN (Long Range Wide Area Network), which is part of the broader category of Low Power Wide Area Networks (LPWAN). ADR optimizes the data transmission rate, power consumption, and airtime of devices based on network conditions, device power capacity, and the quality of the radio link. Here’s how ADR functions and its importance:
- Optimization of Data Rate: ADR dynamically adjusts the data rate at which a device transmits. This is done by changing the spreading factor, bandwidth, and coding rate. Higher data rates can be used when the device is near a gateway, while lower data rates are used as the device moves further away.
- Power Efficiency: By adjusting the data rate, ADR also helps in conserving the battery life of devices. Devices that are closer to a gateway and can transmit at higher data rates will use less power, thus preserving battery life.
- Network Capacity Management: ADR helps in managing the capacity of the network. By ensuring that devices use the optimal data rate, it reduces the time on air for each transmission. This efficiency is crucial in LPWANs as it increases the overall capacity of the network to handle more devices.
- Adaptation to Changing Conditions: ADR responds to changing environmental conditions or changes in the location of the device. If a device’s transmissions start failing, ADR can lower the data rate to increase the chance of successful transmission.
- Manual Override: In some systems, ADR can be manually overridden. This is useful in scenarios where the network administrator knows the environment and can set the data rate to a fixed value for optimal performance.
- Use in LoRaWAN: In LoRaWAN, ADR is a critical feature, especially considering the varying distances between end devices and gateways and the need for long battery life in IoT applications.
- Limitations: ADR is not always suitable for devices that are mobile or experience rapidly changing RF conditions, as it may not react quickly enough to these changes.
In summary, Adaptive Data Rate is a key feature in wireless communication protocols like LoRaWAN, enhancing network efficiency, power consumption, and overall performance of the connected devices. It is particularly important in scenarios where devices must operate over extended periods on limited power sources, such as in many IoT applications.
GSM (Global System for Mobile Communications) is a standard developed by the European Telecommunications Standards Institute (ETSI) to describe the protocols for second-generation (2G) digital cellular networks used by mobile devices such as phones and tablets. Introduced in the 1990s, GSM was a major leap in mobile communication technology. Key aspects of GSM include:
- Digital Communication: GSM marked the transition from analog first-generation (1G) networks to digital, significantly improving voice quality, security, and capacity.
- Global Standard: As its name suggests, GSM became a global standard for mobile communication, facilitating international roaming and compatibility.
- Network Components: GSM networks consist of key subsystems like the Base Station Subsystem (BSS), Network and Switching Subsystem (NSS), and the Operations and Support Subsystem (OSS).
- SIM Cards: GSM introduced the use of SIM (Subscriber Identity Module) cards, which store subscriber data and facilitate mobile device identification and authentication on the network.
- Data Services: Besides voice communication, GSM supports data services such as SMS (Short Message Service) and later, GPRS (General Packet Radio Services) for basic internet connectivity.
- Encryption and Security: GSM networks employ encryption to secure voice and data communication, enhancing privacy and security.
- Frequency Bands: GSM operates in multiple frequency bands, like 900 MHz and 1800 MHz in Europe and 850 MHz and 1900 MHz in the Americas, catering to different regional requirements.
GSM set the foundation for modern mobile communication and led to the development of more advanced technologies like 3G (UMTS) and 4G (LTE).
Wi-Fi HaLow, designated as 802.11ah, is a wireless networking protocol developed by the Wi-Fi Alliance. It’s a part of the IEEE 802.11 set of WLAN standards, but it differs significantly from most of its predecessors. Here are some key aspects of Wi-Fi HaLow:
- Frequency Band: Wi-Fi HaLow operates in the sub-1 GHz spectrum, specifically in the 900 MHz band. This is a lower frequency compared to the 2.4 GHz and 5 GHz bands used by most Wi-Fi technologies. The lower frequency allows for better range and material penetration.
- Range and Coverage: One of the most significant benefits of Wi-Fi HaLow is its extended range. It can cover roughly double the distance of conventional Wi-Fi, making it ideal for reaching into areas that were previously difficult to cover.
- Penetration: The lower frequency also allows for better penetration through obstacles like walls and floors, making Wi-Fi HaLow more reliable in challenging environments.
- Power Efficiency: Wi-Fi HaLow is designed to be more power-efficient, which is crucial for Internet of Things (IoT) devices that often run on batteries. This efficiency extends the battery life of connected devices.
- IoT Applications: Due to its range, penetration, and power efficiency, Wi-Fi HaLow is particularly well-suited for IoT applications, especially in scenarios where devices need to be connected over larger areas or in challenging environments, like smart homes, agricultural settings, industrial sites, and smart cities.
- Device Connectivity: It supports a larger number of connected devices over a single access point compared to traditional Wi-Fi, which is beneficial for IoT environments where many devices need to be connected.
- Security and IP Support: Wi-Fi HaLow retains the high levels of security and native IP support that are characteristic of traditional Wi-Fi standards.
In summary, Wi-Fi HaLow extends the benefits of Wi-Fi to IoT applications, offering solutions to the unique challenges posed by the need for long-range, low-power, high-penetration wireless connectivity. It’s particularly relevant as the number of IoT devices continues to grow, requiring new solutions for connectivity.
LoRaWAN (Long Range Wide Area Network) is a protocol for low-power wide-area networks (LPWANs), designed to wirelessly connect battery-operated ‘things’ to the internet in regional, national, or global networks. It’s particularly useful for the Internet of Things (IoT) applications. Here are some key characteristics and aspects of LoRaWAN:
- Long Range Communication: LoRaWAN is known for its long-range capabilities, often reaching several kilometers in rural areas and penetrating dense urban or indoor environments.
- Low Power Consumption: Devices using LoRaWAN are designed to be power-efficient, which is critical for IoT applications where devices often run on batteries and need to operate for extended periods without maintenance.
- Secure Communication: LoRaWAN includes end-to-end encryption, ensuring secure data transmission, which is crucial in many IoT applications.
- Low Bandwidth: LoRaWAN is optimized for low data rate applications. It’s not suitable for large amounts of data or high-speed communication but is ideal for applications that only need to send small amounts of data over long intervals.
- Star-of-Stars Network Topology: In LoRaWAN networks, gateways relay messages between end-devices and a central network server. The gateways are connected to the network server via standard IP connections, while end-devices use single-hop wireless communication to one or many gateways.
- Adaptive Data Rate (ADR): LoRaWAN can optimize data rates and RF output to balance power consumption, airtime, and network capacity.
- Applications: It’s used in a variety of applications, including smart meters, smart agriculture, smart cities, and environmental monitoring.
- Network Architecture: The architecture is typically laid out in a hierarchical topology to enhance scalability and battery life for end-devices.
- License-Free Frequency Band: LoRaWAN operates in license-free bands such as the industrial, scientific, and medical (ISM) radio bands.
LoRaWAN is an essential technology for IoT ecosystems, especially in scenarios where devices need to communicate over long distances, consume minimal power, and send small amounts of data.
Low Power Wide Area Networks (LPWAN) are a type of wireless telecommunication network designed to allow long-range communications at a low bit rate among connected devices, typically used for M2M (Machine to Machine) and IoT (Internet of Things) applications. Key characteristics and advantages of LPWAN include:
- Long Range: LPWAN technologies are designed to provide wide-area coverage, often covering a radius of several kilometers, even in challenging environments such as urban or industrial areas.
- Low Power Consumption: Devices connected via LPWAN are optimized for low power consumption, which allows them to operate for years on a small battery. This is crucial for IoT applications where devices are often deployed in locations where regular maintenance or battery replacement is not feasible.
- Low Data Rate: LPWAN is optimized for transmissions that require a low data rate. It’s ideal for applications that only need to send small amounts of data intermittently, rather than streaming large quantities of data continuously.
- Cost-Effectiveness: The infrastructure and device costs associated with LPWAN are generally lower compared to other types of wireless networks. This makes LPWAN a practical choice for a wide range of IoT applications.
- Applications: LPWAN is used in a variety of applications, including smart meters, smart agriculture, asset tracking, and environmental monitoring.
- Examples of LPWAN Technologies: Some of the well-known LPWAN technologies include LoRaWAN (Long Range Wide Area Network), NB-IoT (Narrowband IoT), and Sigfox.
In summary, LPWANs play a crucial role in the growth of IoT by connecting devices over long distances with minimal power consumption and lower costs, making it feasible to deploy large networks of sensors and devices.
MIMO (Multiple Input Multiple Output) is a wireless technology used in communication systems, particularly in modern Wi-Fi and cellular networks like LTE and 5G. It involves the use of multiple antennas at both the transmitter and receiver to improve communication performance. Key aspects of MIMO include:
- Increased Data Throughput: By using multiple antennas, MIMO can transmit more data simultaneously compared to systems with a single antenna, significantly increasing the network’s data throughput.
- Spatial Multiplexing: This technique, used in MIMO systems, transmits different data streams simultaneously over the same frequency band but through different spatial paths. It effectively multiplies the capacity of the radio channel.
- Diversity Gain: MIMO can provide diversity gain by transmitting the same data across different antennas, reducing the likelihood of data loss due to fading or interference.
- Improved Signal Quality: MIMO systems can improve signal quality and reduce error rates by combining multiple received signals, which have traveled through different paths and thus experienced different levels of fading and interference.
- Beamforming: Advanced MIMO systems use beamforming to direct the signal towards the intended receiver, enhancing the signal strength and reducing interference to and from other devices.
- Types of MIMO:
- SU-MIMO (Single-User MIMO): Involves one transmitter and one receiver, each with multiple antennas.
- MU-MIMO (Multi-User MIMO): Allows communication with multiple users simultaneously, each with one or more antennas.
- Applications: MIMO technology is a foundational element in modern wireless communication standards, including Wi-Fi (802.11n, ac, ax), LTE, and 5G networks.
MIMO technology represents a significant advancement in wireless communications, enabling more efficient and reliable transmission of data, and is essential for achieving the high-speed and high-capacity requirements of current and future wireless networks.
Open RAN (Open Radio Access Network) is an initiative to create more open and interoperable wireless network architectures. It represents a shift from traditional RAN solutions, which often involve proprietary, integrated systems from a single vendor, to a more modular and flexible approach. Key aspects of Open RAN include:
- Open Interfaces: Open RAN emphasizes the use of standardized, open interfaces between various components of the radio access network. This allows for interoperability between different vendors’ equipment.
- Decoupling Hardware and Software: It enables the decoupling of hardware and software functionalities in the network, allowing operators to mix and match hardware and software from different suppliers.
- Vendor Diversity and Innovation: By promoting open standards and interfaces, Open RAN encourages more vendors to participate in the ecosystem, fostering innovation and potentially reducing costs.
- Virtualization and Software-Defined Networking: Open RAN leverages virtualization technologies and software-defined networking (SDN) principles, leading to more flexible and scalable networks.
- Increased Efficiency and Agility: Networks based on Open RAN can adapt more quickly to changing demands and technologies, improving efficiency and service quality.
- Support for 5G and Beyond: Open RAN is seen as a key enabler for the rollout of 5G networks, offering the agility and scalability needed to support 5G’s diverse use cases.
- Organizations and Alliances: Several industry alliances and organizations, such as the O-RAN Alliance and the Telecom Infra Project (TIP), are driving the development and adoption of Open RAN standards.
Open RAN represents a transformative approach in the deployment and operation of mobile networks, promising to enhance competition, innovation, and flexibility in the telecom industry.
Quadrature Amplitude Modulation (QAM) is a modulation technique used in various forms of communication systems, including digital television and wireless communications. It combines two amplitude-modulated signals into a single channel, thereby increasing the bandwidth efficiency. Here’s a more detailed look at QAM:
- Combining Amplitude and Phase Modulation: QAM works by varying both the amplitude and the phase of a carrier signal. Essentially, it’s a blend of both amplitude modulation (AM) and phase modulation (PM).
- Constellation Diagram: In QAM, data points represented in a modulation are often visualized using a constellation diagram, which plots the amplitude and phase variations as points on a two-dimensional graph. Each point on the diagram represents a different symbol.
- Increased Data Rates: By varying both amplitude and phase, QAM can transmit more data per symbol compared to using either modulation technique alone. This makes it more bandwidth-efficient and enables higher data transmission rates.
- Applications in Digital Transmission: QAM is widely used in digital radio and television broadcasting, cable TV systems, and in some wireless communication systems like Wi-Fi and cellular networks.
- Variants of QAM: There are several variants of QAM, like 16-QAM, 64-QAM, 256-QAM, and others. The number denotes how many different symbols can be represented; for instance, 256-QAM can represent 256 different symbols. Higher QAM levels can transmit more bits per symbol, but they also require a higher signal-to-noise ratio to avoid errors.
- Adaptive QAM: In some communication systems, QAM can be adaptively changed depending on the channel conditions. For example, a system might use a higher level of QAM when signal conditions are good and a lower level when they are less favorable to maintain the quality of the transmission.
- Challenges with Higher QAM Levels: As the level of QAM increases, the spacing between constellation points becomes tighter, making the system more susceptible to noise and errors. Hence, higher QAM levels require better quality transmission channels.
In summary, QAM is a fundamental modulation technique that enables efficient use of available bandwidth by combining amplitude and phase modulation, widely used in modern digital communication systems.
Spectrum licensing refers to the regulatory process whereby national governments or regulatory bodies authorize the use of specific parts of the radio frequency spectrum by individuals, companies, or organizations. This process is crucial for managing the radio spectrum, which is a finite resource. Key aspects of spectrum licensing include:
- Regulatory Authority Involvement: Spectrum licensing is typically overseen by a national regulatory authority, such as the Federal Communications Commission (FCC) in the United States or Ofcom in the United Kingdom.
- Allocation and Assignment: The process involves allocating frequency bands for specific uses (such as mobile communication, broadcasting, or satellite transmission) and assigning specific frequencies or bands to licensees.
- License Types: There are various types of spectrum licenses, including exclusive use licenses, shared use licenses, and unlicensed spectrum allocations (like the bands used for Wi-Fi).
- Auctioning Spectrum: Many countries use auctions to allocate spectrum licenses, allowing companies to bid on the rights to use certain frequency bands. This method is often used for commercial purposes like mobile networks.
- Licensing Fees: Licensees typically pay a fee for spectrum use rights. Fees can vary based on the spectrum band, the geographic coverage of the license, and the duration of the license.
- Conditions and Regulations: Spectrum licenses come with conditions and regulations to ensure efficient and non-interfering use of the spectrum, including technical specifications, usage limitations, and compliance with international agreements.
- Spectrum Management: Effective spectrum licensing is a critical aspect of spectrum management, ensuring that this valuable resource is used efficiently and in a way that minimizes interference between different users.
- Economic and Strategic Importance: Spectrum licensing is not only a regulatory process but also of significant economic and strategic importance, influencing the development and deployment of wireless communication technologies.
Spectrum licensing is a key tool in the management of radio frequencies, balancing the need for efficient use of the spectrum, technological innovation, and economic considerations.
Spectrum Reallocation Strategy refers to the process of reassigning and repurposing frequency bands for different uses, typically within the context of wireless communications. This strategy is crucial in managing the finite resource of the radio spectrum, especially with the increasing demand for wireless services. Key aspects of Spectrum Reallocation Strategy include:
- Addressing Spectrum Scarcity: With the growing number of wireless devices and services, such as mobile phones, IoT devices, and broadband services, the demand for radio spectrum has significantly increased, leading to the need for efficient spectrum management.
- Reallocating for New Technologies: As new technologies like 5G emerge, reallocating spectrum bands to accommodate these technologies becomes necessary to ensure they have the necessary bandwidth to operate effectively.
- Balancing Interests: The process involves balancing the needs and interests of various stakeholders, including government agencies, private sector companies, and the public.
- Regulatory Decisions: National and international regulatory bodies, such as the Federal Communications Commission (FCC) in the U.S. or the International Telecommunication Union (ITU) globally, play a key role in making decisions about spectrum reallocation.
- Auctioning Spectrum: Often, reallocated spectrum is auctioned off to the highest bidder, providing a transparent and market-driven mechanism for allocation.
- Minimizing Disruption: Careful planning is required to minimize disruption to existing services and users in bands that are being reallocated.
- Economic Implications: Spectrum reallocation can have significant economic implications, both in terms of the revenue generated from spectrum auctions and the economic benefits of new technologies and services that use the reallocated spectrum.
Spectrum Reallocation Strategy is a critical aspect of modern telecommunications policy, ensuring that this valuable resource is used effectively to meet current and future needs.
A “License-Free Frequency Band” refers to parts of the radio spectrum that can be used without the need to acquire a license from regulatory authorities. These bands are open for public use under certain regulations and guidelines set by the governing bodies, like the Federal Communications Commission (FCC) in the United States or similar organizations in other countries. Key features and implications of these bands include:
- Open Access: Individuals and companies can operate devices in these bands without needing to obtain a license, which lowers the barriers to entry for developing and deploying wireless technologies.
- Regulatory Guidelines: Although no license is required, there are still regulations that govern the use of these bands. These typically include limits on transmission power, requirements for equipment to tolerate interference, and rules to minimize the risk of devices interfering with each other.
- Common Uses: License-free bands are commonly used for consumer wireless devices like Wi-Fi routers (in the 2.4 GHz and 5 GHz bands), Bluetooth devices, cordless telephones, and other short-range communication devices. They’re also used for industrial, scientific, and medical (ISM) applications.
- Popular License-Free Bands: The most well-known license-free bands are the ISM bands, which include frequencies around 900 MHz, 2.4 GHz, and 5 GHz. These bands are widely used for a variety of wireless communication technologies.
- Advantages: The primary advantage of using license-free bands is the reduced cost and complexity associated with bringing a wireless product to market. There’s no need to bid for spectrum rights or pay licensing fees.
- Challenges: A major challenge in these bands is the potential for interference, as many different devices and technologies may be operating in the same frequency range. This can impact the performance and reliability of wireless communications.
- Global Variation: The availability and specific regulations of license-free bands can vary from one country to another, so manufacturers need to ensure their devices comply with the regulations in each market where they are sold.
In summary, license-free frequency bands are crucial for a wide range of wireless technologies, especially for consumer and small-scale industrial applications. They enable easier access to the radio spectrum but come with the responsibility of adhering to regulations and managing interference.
Wi-Fi 4, officially known as IEEE 802.11n, is the fourth generation of Wi-Fi standards and was a significant advancement over the previous Wi-Fi standards, particularly IEEE 802.11g. Introduced in 2009, Wi-Fi 4 brought several key improvements to wireless networking:
- Increased Speed: Wi-Fi 4 offered higher maximum data rates, up to 600 Mbps under ideal conditions, which was a substantial improvement over the 54 Mbps maximum of its predecessor.
- MIMO Technology: Wi-Fi 4 introduced Multiple Input Multiple Output (MIMO) technology. This allowed the use of multiple antennas for both transmission and reception, enhancing data throughput and signal range.
- Dual-Band Operation: Wi-Fi 4 could operate on both the 2.4 GHz and 5 GHz bands, giving users the flexibility to choose the band with less interference and better performance.
- Wider Channel Bandwidth: It supported channel bandwidths of up to 40 MHz, wider than the 20 MHz channels of previous standards. This allowed for more data to be transmitted simultaneously.
- Improved Range and Reliability: The range and reliability of Wi-Fi connections were significantly improved, offering better performance at greater distances and in environments with physical obstructions.
- Backward Compatibility: Wi-Fi 4 was backward compatible with earlier Wi-Fi standards, ensuring that devices supporting older standards could still connect to Wi-Fi 4 networks.
Wi-Fi 4 played a crucial role in advancing wireless networking technology, facilitating faster speeds, increased range, and better overall performance, paving the way for the development of subsequent Wi-Fi generations.
Wi-Fi 5, known technically as IEEE 802.11ac, is the fifth generation of Wi-Fi standards. It was a significant improvement over its predecessor, Wi-Fi 4 (802.11n), and brought several advancements:
- Higher Data Rates: Wi-Fi 5 offers greater maximum data rates than Wi-Fi 4, primarily due to more efficient data encoding. This results in faster speeds for users.
- Dual-Band Operation: Unlike Wi-Fi 4, which primarily operates on the 2.4 GHz band, Wi-Fi 5 operates on both 2.4 GHz and 5 GHz bands. The 5 GHz band offers less interference and higher speeds.
- Wider Channel Bandwidth: Wi-Fi 5 supports wider channel bandwidths of up to 160 MHz (compared to the maximum of 40 MHz in Wi-Fi 4), allowing more data to be transmitted simultaneously.
- MU-MIMO (Multi-User, Multiple Input, Multiple Output): This technology enables a Wi-Fi router to communicate with multiple devices at the same time, increasing network efficiency and throughput.
- Beamforming: Beamforming technology in Wi-Fi 5 helps in focusing the Wi-Fi signal towards the device, rather than broadcasting it in all directions, which enhances the signal strength and reliability.
- Backward Compatibility: Wi-Fi 5 is backward compatible with previous Wi-Fi standards, ensuring that older devices can still connect to Wi-Fi 5 networks.
Wi-Fi 5 represented a substantial step forward in wireless networking technology, offering improved speeds, efficiency, and capacity, particularly for environments with high data demands.
Wi-Fi 6, officially known as IEEE 802.11ax, is the sixth generation of Wi-Fi standards and a significant upgrade over its predecessor, Wi-Fi 5 (802.11ac). Introduced to provide better performance in environments with a lot of connected devices, Wi-Fi 6 offers several improvements:
- Increased Data Rates: Wi-Fi 6 provides higher data rates compared to Wi-Fi 5, thanks to more efficient data encoding and larger channel bandwidth. This results in faster internet speeds and better performance.
- Improved Network Efficiency: One of the key features of Wi-Fi 6 is OFDMA (Orthogonal Frequency Division Multiple Access), which allows one transmission to deliver data to multiple devices at once. This significantly improves efficiency, especially in crowded networks.
- Better Performance in Congested Areas: Wi-Fi 6 shines in areas with many connected devices, such as stadiums, airports, and urban apartments. It reduces latency and improves throughput, making the network more responsive.
- Enhanced Battery Life for Connected Devices: Wi-Fi 6 introduces Target Wake Time (TWT), a feature that schedules communication between the router and devices. This reduces the amount of time devices need to keep their antennas active, conserving battery life.
- Improved Security: Wi-Fi 6 comes with WPA3, the latest Wi-Fi security protocol, which enhances user data protection, especially on public networks.
- Backward Compatibility: Wi-Fi 6 routers and devices are backward compatible with previous Wi-Fi standards, ensuring that older devices can still connect to new networks.
- Wider Channel Bandwidth: It supports 1024-QAM (Quadrature Amplitude Modulation), which increases throughput for emerging, bandwidth-intensive use cases.
- MU-MIMO Enhancements: Multi-user, multiple input, multiple output (MU-MIMO) technology allows more data to be transferred at once and enables an access point to communicate with more than one device simultaneously.
Wi-Fi 6 is designed for the next generation of connectivity, offering faster speeds, greater capacity, and better performance in environments with a lot of wireless devices.
Wi-Fi 7, technically known as 802.11be, is the forthcoming generation of Wi-Fi technology, following Wi-Fi 6 (802.11ax). It is being developed by the IEEE (Institute of Electrical and Electronics Engineers) and is expected to significantly enhance wireless networking performance. Key features and advancements of Wi-Fi 7 include:
- Higher Data Rates: Wi-Fi 7 is anticipated to offer substantially higher maximum data rates than Wi-Fi 6, potentially up to 30-40 Gbps. This is achieved through increased bandwidth and more efficient use of the wireless spectrum.
- Enhanced Bandwidth Utilization: The standard aims to support wider channel bandwidths, up to 320 MHz, and improve the utilization of available frequency bands, including 2.4 GHz, 5 GHz, and 6 GHz.
- Multi-Link Operation (MLO): A significant feature of Wi-Fi 7, MLO allows devices to use multiple bands and channels simultaneously. This can enhance throughput, reduce latency, and improve reliability.
- Advanced Modulation Techniques: Wi-Fi 7 is expected to support 4096-QAM (Quadrature Amplitude Modulation), enabling more bits to be transmitted with each signal, thus increasing the overall data throughput.
- Improved Latency: The new standard aims to significantly reduce latency, which is crucial for applications that require real-time communication, such as online gaming, augmented reality, and virtual reality.
- Increased Network Efficiency: Wi-Fi 7 is designed to be more efficient, especially in environments with many active devices, by utilizing more sophisticated technologies like improved spatial reuse and better scheduling algorithms.
- Backward Compatibility: Like its predecessors, Wi-Fi 7 is expected to be backward compatible with older Wi-Fi standards, ensuring a smooth transition for users upgrading their network hardware.
- Better Power Management: Features like Target Wake Time (TWT) are likely to be enhanced to improve power efficiency for IoT and mobile devices, extending their battery life.
Wi-Fi 7, with its advanced capabilities, is poised to meet the growing demands for higher data rates, lower latency, and more efficient networking in increasingly crowded and diverse wireless environments. As of my last update in April 2023, Wi-Fi 7 is still in the development phase, with its finalization and widespread adoption expected in the following years.
Wi-Fi HaLow, designated as 802.11ah, is a wireless networking protocol developed under the IEEE 802.11 standard. It’s specifically designed for the Internet of Things (IoT) applications. Key features and aspects of Wi-Fi HaLow include:
- Sub-GHz Operation: Unlike traditional Wi-Fi that operates in the 2.4 GHz and 5 GHz bands, Wi-Fi HaLow operates in frequency bands below 1 GHz. This allows for better range and penetration through obstacles like walls and floors.
- Extended Range: Wi-Fi HaLow is known for its long-range capabilities, typically offering coverage over several kilometers. This makes it ideal for IoT applications spread over large areas, like agricultural or industrial environments.
- Low Power Consumption: Devices using Wi-Fi HaLow are designed for low power usage, which is essential for IoT devices, many of which need to operate for years on a small battery.
- High Device Capacity: Wi-Fi HaLow can support thousands of connected devices under a single access point, much more than traditional Wi-Fi. This is particularly important for IoT applications, where many devices are often deployed in a condensed area.
- Use Cases: Wi-Fi HaLow is suited for a range of IoT applications, including smart home and building automation, agricultural and environmental sensors, and industrial monitoring.
- Compatibility and Security: Wi-Fi HaLow retains the core characteristics of the Wi-Fi protocol, including security protocols and ease of integration with existing Wi-Fi technologies.
- Data Rates: While it supports lower data rates compared to conventional Wi-Fi, it’s sufficient for the typical data needs of IoT devices, which usually transmit small amounts of data.
In summary, Wi-Fi HaLow extends the versatility of Wi-Fi to IoT applications, offering solutions for long-range, low-power, and high-density connectivity challenges.